Difference between revisions of "Wiki-INPRO-methodology"
Line 191: | Line 191: | ||
*Emergency heat removal system is based on the passive removal of heat from the reactor to the environment through the sodium-sodium and sodium-air heat exchangers, and designed in such a way that in the case of emergency cooling the primary sodium circulates through the reactor core fuel assemblies driven by natural circulation flow; | *Emergency heat removal system is based on the passive removal of heat from the reactor to the environment through the sodium-sodium and sodium-air heat exchangers, and designed in such a way that in the case of emergency cooling the primary sodium circulates through the reactor core fuel assemblies driven by natural circulation flow; | ||
*The above the reactor premise is used for confinement of radioactive gases and aerosols prior to subsequent filtration in the special ventilation system reducing the radioactivity of released gases. Calculated core damage frequency of the BN-1200 reactor (approx. 5×10<sup>-7</sup> 1/a) is essentially reduced compared with those of BN-800 (approx. 2×10<sup>-6</sup> 1/a) and BN-600 (approx. 10<sup>-5</sup> 1/a). | *The above the reactor premise is used for confinement of radioactive gases and aerosols prior to subsequent filtration in the special ventilation system reducing the radioactivity of released gases. Calculated core damage frequency of the BN-1200 reactor (approx. 5×10<sup>-7</sup> 1/a) is essentially reduced compared with those of BN-800 (approx. 2×10<sup>-6</sup> 1/a) and BN-600 (approx. 10<sup>-5</sup> 1/a). | ||
+ | |||
+ | ====INPRO sustainability assessment of BN-1200 in the area of economics==== | ||
+ | This section presents an overview of the assessment method used in the INPRO area of economics and modifications to the method that have been introduced in the study. It provides input parameters used in the calculations and basic assumptions made by the national experts performing such calculations. Finally, the results of calculations presented in this section may be considered as an input for further research activities focused on optimization of the fast reactors designs and fuel cycles, however they should not be considered in relation to any commercial activities. | ||
+ | =====Overview of the application of the INPRO methodology area of economics to the fast reactors under development===== | ||
+ | The INPRO methodology has been developed for the sustainability assessment of the nuclear energy systems comprising different types of reactors and fuel cycle facilities. Sustainability assessment using INPRO methodology covers several different areas including economics, reactor safety, safety of fuel cycle facilities, waste management, etc. However, in the area of economics the INPRO methodology focuses on economic characteristics of energy products, i.e. electricity, heat, etc, generated by an NPP, rather than on economic parameters of fuel cycle facilities which are used as an input in a form of ‘cost of a given service’. For example, unless such calculations are the only way of obtaining costs of services the assessor is not required to calculate the figures of merit of the enrichment or reprocessing facility for the purpose of INPRO sustainability assessment. Instead, the costs of enrichment or reprocessing services are aggregated along with other costs in the figures of merit of the NPP producing final energy product. These figures of merit are used for the assessment against INPRO criteria in the area of economics.<br> | ||
+ | INPRO methodology recommendations have the same hierarchy in all areas of assessment. On top of this structure a basic principle of sustainability is defined for every area determining a goal to be achieved to make the nuclear energy system sustainable<ref name=r13>INTERNATIONAL ATOMIC ENERGY AGENCY, INPRO Methodology for Sustainability Assessment of Nuclear Energy Systems: Economics, IAEA Nuclear Energy Series No. NG-T-4.4, IAEA, Vienna (2014).</ref>. The necessary actions to be taken to achieve the goal defined in the basic principle are introduced on the second level and called ‘user requirements’. At the next and third level, every user requirement splits into a few criteria. Criteria are the tools for assessment. Every criterion consists of an indicator (e.g. parameter) and an acceptance limit defining the range of values satisfying this criterion.<br> | ||
+ | The INPRO assessment is normally done on the criteria level. When all criteria comprising a given user requirement are met, it means that necessary action occurred in a correct manner to meet the user requirement. When all given user requirements in a given area are met, the goal defined in the corresponding INPRO basic principle is also met<ref name=r13>INTERNATIONAL ATOMIC ENERGY AGENCY, INPRO Methodology for Sustainability Assessment of Nuclear Energy Systems: Economics, IAEA Nuclear Energy Series No. NG-T-4.4, IAEA, Vienna (2014).</ref>. However, the INPRO methodology assessment is not only used for the confirmation of nuclear energy system sustainability. The INPRO assessment criteria which are not met provide valuable information on the gaps in the nuclear power programme and help to define follow-up actions (e.g. R&D) necessary to close these gaps. Comparison of the different assessment results may help to estimate the potential advantages of the assessed nuclear energy systems.<br> | ||
+ | Judgement on the system sustainability can be derived from the results of full scope INPRO assessment in all INPRO methodology areas. However, preliminary results of the assessment in selected areas can be used for the optimization of nuclear energy system or modification of the selected system components. Although such limited scope assessments can provide valuable information on the system sustainability, the judgement on sustainability can be concluded only in the full scope INPRO sustainability assessment study in all areas.<br> | ||
+ | This limited scope assessment study omits INPRO areas involving the essential numbers of country specific criteria (waste management, infrastructure, proliferation resistance) and focuses on the two areas encompassing the most of design related information – economics and reactor safety.<br> | ||
+ | The INPRO methodology in the area of economics sets up the goal of achieving ‘affordable and available’ produced energy and related products and services in a sustainable nuclear energy system<ref name=r13>INTERNATIONAL ATOMIC ENERGY AGENCY, INPRO Methodology for Sustainability Assessment of Nuclear Energy Systems: Economics, IAEA Nuclear Energy Series No. NG-T-4.4, IAEA, Vienna (2014).</ref>. The affordability of energy is understood as cost competitiveness with alternatives available in the country or region. The availability of nuclear energy is seen as the ability to finance the project at acceptably low investment risk.<br> | ||
+ | Four user requirements have been introduced in the INPRO methodology area of economics to specify how the basic principle can be met<ref name=r13>INTERNATIONAL ATOMIC ENERGY AGENCY, INPRO Methodology for Sustainability Assessment of Nuclear Energy Systems: Economics, IAEA Nuclear Energy Series No. NG-T-4.4, IAEA, Vienna (2014).</ref>: | ||
+ | *The cost of energy supplied by nuclear energy systems, taking all relevant costs and credits into account, should be competitive with that of alternative energy sources, that are available for a given application in the same time frame and geographic region/jurisdiction; | ||
+ | *The total investment required to design, construct, and commission nuclear energy systems, including interest during construction, should be such that the necessary investment funds can be raised; | ||
+ | *The risk of investment in nuclear energy systems should be acceptable to investors; | ||
+ | *Innovative nuclear energy systems should be compatible with meeting the requirements of different markets. | ||
+ | The developer of sustainable energy technology is expected to meet these user requirements. The INPRO assessor has eight criteria which can be used for checking the status of nuclear energy system in relation to the INPRO user requirements: | ||
+ | *Cost competitiveness; | ||
+ | *Attractiveness of investment; | ||
+ | *Investment limit; | ||
+ | *Maturity of design; | ||
+ | *Construction schedule; | ||
+ | *Uncertainty of economic input parameters; | ||
+ | *Political environment; | ||
+ | *Flexibility. | ||
+ | Only five of these eight criteria have been assessed in this study as explained below. The cost competitiveness criterion uses the cost of energy generated by the nuclear energy system as an indicator, i.e. characteristic to be assessed. The limit for acceptance of this characteristic was defined as: | ||
Revision as of 15:09, 24 August 2022
The INPRO methodology is an IAEA tool that assists Member States in strategic planning and decision making on nuclear power programmes by sustainability assessment of a NES.
Contents
- 1 Introduction of the INPRO methodology
- 2 Application of the INPRO methodology
- 2.1 Limited Scope Sustainability Assessment of Planned Nuclear Energy Systems Based on BN-1200 fast reactors
- 2.2 INPRO Assessment of the Planned Nuclear Energy System of Belarus
- 3 References
Introduction of the INPRO methodology
Application of the INPRO methodology
Limited Scope Sustainability Assessment of Planned Nuclear Energy Systems Based on BN-1200 fast reactors
Introduction
Objective
This publication provides an example of the limited scope INPRO sustainability assessment of an innovative nuclear energy system using the fast reactor BN-1200 as a case study. The INPRO assessment performed at the full depth criteria level helped to identify actions, including potential research development and demonstration, for sustainable long term deployment of sodium cooled fast reactors.
This publication discusses the application of the INPRO sustainability assessment method to the innovative nuclear energy system based on fast reactor BN-1200 in the areas of economics and safety of nuclear reactors. The case study is intended to verify readiness of the updated INPRO Methodology for assessment of the sodium cooled fast reactors and to develop recommendations for further improvements and updates of the INPRO assessment method.
This publication is intended for use by organizations involved in the development and deployment of the nuclear energy systems including planning, design, modification, technical support and operation for nuclear power plants. Data provided in this publication can be used in further detailed INPRO sustainability assessments of the nuclear energy systems based on BN-1200 reactors, sustainability assessments of other fast reactors and in scenario modelling studies involving fast reactors which can be carried out by the technology holders and technology users.
Scope
Limited scope INPRO sustainability assessment of sodium cooled fast reactors was performed in 2015-2019 in parallel as a series of bilateral studies between the developers of fast reactors and the IAEA in a few countries developing such reactors. Every study was conducted as a self-assessment exercise performed by the national designer experts focused on their own design and supported by the IAEA staff.
This publication presents the results of the case study of the INPRO assessment of BN-1200 reactor in the INPRO areas of economics and reactor safety. The BN-1200 assessment has been performed by the Russian Federation experts from the Institute of Physics and Power Engineering with the support provided by JSC Afrikantov OKB Mechanical Engineering. It is based on assessors’ experience and publicly available data, taking into account proprietary information concerns.
This INPRO methodology sustainability assessment study is focused on the nuclear power plants that produce primarily electricity, heat or combinations of the two. This publication does not explicitly consider economics and safety issues related to other non-electric applications (hydrogen production, desalination, etc.) or to cogeneration involving such energy products. It is expected that as more detailed information is acquired on the safety of interactions between a reactor and industrial facilities located on the same site, the INPRO criteria and the assessment studies may be modified accordingly.
Structure
This publication follows the relationship between the concept of sustainable development and INPRO methodology areas of economics and reactor safety. Section 2 provides general information on the fast reactor development programme in the Russian Federation, to set the context. Section 3 presents the INPRO sustainability assessment of BN-1200 in the area of economics. This includes an overview of the application of the INPRO methodology area of economics to the fast reactors under development, information on the improvement of economic characteristics of sodium cooled fast reactors in the Russian Federation, basic results of analysis and sustainability assessment of BN-1200 in the area of economics. Section 4 presents the INPRO sustainability assessment on the criterion level in the area of reactor safety including assessment of the design robustness, detection and interception of anticipated operational occurrences, design basis accidents, severe plant conditions, independence of levels of defence in depth, inherent safety characteristics, passive safety systems, human factors related to safety and necessary research, development and demonstration. Section 5 summarises the discussion and suggests conclusions on the performed study.
General information on the fast reactors considered in the assessment study
Liquid metal cooled fast reactors development programme in the Russian Federation involves the following commercial sodium cooled fast reactors of BN lineage:
- BN-350 prototype reactor constructed and operated (1973-1999) in Kazakhstan;
- BN-600 reactor operated since 1980 as unit 3 of the Beloyarsk NPP, Russian Federation;
- BN-800 reactor operated since 2016 as unit 4 of the Beloyarsk NPP, Russian Federation;
- BN-1200 reactor design; pilot unit is planned to be constructed at the Beloyarsk NPP site as unit 5.
Besides that, this programme includes two more types of the liquid metal cooled fast reactors:
- Lead-cooled fast reactor design concept BREST- OD-300 with on-site manufacturing of nuclear fuel and reprocessing of spent nuclear fuel;
- Lead-bismuth cooled reactor SVBR-100 (installed electrical power rating – 100 MW(e)) which is being developed under the public-private partnership framework.
The associated closed nuclear fuel cycle facilities / technologies have been developed in the Russian Federation:
- MOX fuel experimental fuel assemblies tested in BN-350 and BN-600 reactors (approx. 50 bundles with pellet fuel and approx. 30 bundles with vibro-packed fuel);
- Mining and Chemical Combine (GHK) commercial MOX fuel fabrication facility for BN-800;
- RT-1 spent fuel reprocessing facility (PUREX technology) for uranium oxide fuel from VVER-440 (water cooled reactors) and BN-600;
- Experimental technology for the nitride fuel fabrication;
- Laboratory level technology for the pyrochemical reprocessing of irradiated nuclear fuel.
This INPRO sustainability assessment focuses on BN-1200 reactor as an example case study. In the area of reactor safety, the BN-800 reactor was selected as a reference design for BN-1200 and in a few cases (e.g. references to the accrued operational experience) the BN-600 reactor data have been used. In the INPRO area of economics the BN-800 and BN-600 reactor data have been used for estimation of trends in economic characteristics of BN reactors.
General information on BN-600, -800, -1200 reactors is presented in this section for broader context of the study.
BN-600 reactor
The original plan for the development of BN-600 reactor was based on the following basic assumptions:
- BN-600 would have higher steam temperature and pressure (540°C, 140 MPa) and increased electrical power rating compared to BN-350;
- The original basic concept and layout of BN-600 reactor were originally expected to be similar to BN-350 (loop type reactor);
- The highest electrical power rating of the standard turbo-generators designed for these parameters of steam and available at that time was 200 MW(e). The electrical power rating of BN-600 was defined as 3×200 MW(e). The reactor concept used three completely independent heat transport loops in the secondary and tertiary circuits.
The BN-600 design process modified the original conceptual ideas. Hence, the BN-600 reactor constructed at Beloyarsk NPP site contains significant improvements[1] in comparison to the original requirements.
Loop type reactor designs have been broadly used in many countries at the early stages of development of the fast reactors. However, as sodium cooled fast reactor designs grew in installed power rating a loop type layout was revealed to have a number of complicated engineering problems.
Loop type fast reactor designs used relatively long pipelines of large cross section connecting the reactor vessel and intermediate heat exchangers. High temperature and radioactive primary sodium circulating in these pipelines change temperature at changes of reactor power level and creates essential tension/stress loads on the pipes and welds. Compensation of the thermal expansions by bending of the pipelines increases the primary circuit piping length and impedes the primary coolant natural circulation which is important for reactor cooling under the postulated station blackout conditions. Besides that, this does not provide an efficient solution since the mechanical stress in bends may become very close to the yield stress.
Moreover, the main pipeline tension/stress loads compensation forces propagate to the relatively thin walled casings of the pipes, vessels and nozzles occasionally deteriorating their stress-strain characteristics. The areas of the reactor nozzles seemed to be the most vulnerable parts and belonged to the group of most challenging components to fabricate since the stresses caused by the reactor coolant parameter cycles in these locations were the most frequent and the ranges of changing values were broad. Eliminating the nozzles provides an inherent safety feature associated with this hazard and seemed to be the most effective way to increase the robustness of the reactor design. Hence, a high power rating pool type reactor design seemed to have more reliability than a loop type designs.
The electric heating system associated with the primary coolant pipelines and the systems connected to the primary circuit of a loop type reactor had to involve sophisticated and expensive components for large isolating valves and casings of the pipes that cannot be isolated in the case of a leak. This equipment aimed to rule out any dangerous decrease in the reactor sodium level in case of the coolant leakage through the failed pipeline.
More challenges occur implementing fire and radiation protection from the sodium leaks, in particular those caused by the guillotine break of the primary pipeline – an accident scenario postulated to be accounted for by the national regulatory requirements.
Pool type layout has significant advantages compared against loop type sodium cooled fast reactors. The main reactor vessel design can avoid pipeline connections under the normal operation sodium level and accommodation of the primary circuit systems structures and components within the reactor vessel essentially reduces the probability of radioactive sodium leaks to the confinement/ containment premises and makes the solution of leak-tightness problems much easier. The reactor vessel walls, bottom and support structures are designed to withstand mechanical forces caused by the weight of reactor vessel, sodium weight and the weight of reactor internals and fuel. In the pool type design, forces coming from the thermal expansion of reactor pipelines and stressing the reactor vessel nozzles do not exist.
Placing the primary circuit systems, structures and components inside of the reactor vessel reduces the surface-to-volume ratio of the radioactive sodium and the length of welds where in the case of failure a leakage may occur. In addition to the apparently positive safety effects the cost of materials and the reactor fabrication efforts reduce significantly.
In the pool type fast reactor, accommodation of the primary circuit equipment within the large volume of sodium increases the system inertia and makes the system parameters more stable. All internals are immersed in sodium and small sodium leaks through the detachable joints between the reactor internals caused by the pressure difference on different sides can be accepted. Some of the walls of systems, structures and components placed inside the reactor vessel are not required to withstand significant strain, unlike those in the loop type reactors, and can be thinner and/or easier to fabricate. Thinner walls of reactor internals further reduce thermal stresses during the reactor transients.
Elimination of primary circuit pipelines or at least effective minimization of their length essentially reduces the cost of materials used, cost of equipment manufacturing and cost of NPP construction. Sealed compartments of the loop type design primary circuit can be eliminated, reducing the associated costs of ventilation and fire-protection systems, costs of electric heating system, costs of guard casings, thermal insulation and drains. The size and costs of several other systems can be essentially reduced, e.g. the biological shielding is required only for the reactor vessel and a few remaining pipelines and systems containing radioactive sodium. The in-vessel neutron shielding installed in the pool type reactors reduces the radiation dose to the reactor vessel and internals. It also allows for in-vessel spent fuel storage.
Another important feature of the pool type reactor is the possibility of passive removal of the residual heat in emergency situations[1]. Passive heat removal depends on natural circulation of the primary and secondary sodium achieved through complex thermal-hydraulic design considerations in the reactor, heat exchangers and steam generators. At the time of BN-600 design development the passive removal of residual heat through the main circuits (three channels) was considered to be reliable.
The deficiencies of pool type designs are mostly related to the size of reactor vessel and to the mass of in-vessel systems, structures and components. Pool type reactor vessels are normally too large to be manufactured at the fabrication facility and transported in one piece to the NPP site. In this situation the reactor vessels need to be manufactured on the site which makes this process more expensive and challenging. The size and mass of reactor can make it vulnerable to the seismic loads and may require special arrangements different from other types of reactors. Other challenges are associated, for example with the introduction of compact and reliable in vessel neutron shielding having sufficiently long lifetime, with the reliable reactor vessel support structure and with the neutron flux monitoring necessary for the NPP power control[1].
Several major concepts have been incorporated in the final design of BN-600 as follows:
- Pool type layout of the primary circuit. This configuration helps to simplify the design, resolve several engineering problems associated with large fast reactors, and provides the designer with conditions and tools which allow both safety improvements and cost reductions;
- New neutron absorbers. New shim rods with higher absorbing efficiency have been installed to compensate fuel burnup effects. It allows an extension of the time between refuelling and improvement of economic characteristics for the plant;
- New design of primary coolant pumps. The primary coolant pumps use a new design with a bottom hydrostatic bearing working under sodium. This design allows control of the pump speed, reduction of the pressure in the reactor vessel gas plenum and further simplification of the layout of the primary and secondary circuits;
- New design of steam generators. Once-through sectional-modular steam generators and sodium-steam reheating scheme provide robustness for the higher temperature and pressures of steam generated in BN-600. In the case of malfunctions of the heat exchanging components the sectional design of steam generators allows isolation of a given section and uninterruptable operation of others;
- Independent heat removal loops in secondary and tertiary circuits. This layout allows for higher flexibility of the operating regimes, e.g. several reactor start-up procedures were performed consecutively and separately in every loop. The reactor can operate at power levels less than 67% of full power using only two pairs of secondary and tertiary loops. Operation of reactor at any power levels with only one pair of secondary and tertiary loops is not allowed.
The BN-600 reactor’s first criticality was achieved in 1980. The power plant unit construction used general civil industrial type building construction requirements. BN-600 has three steam generators PGN-200M, three turbines of the K-200-12.8-3 type and three electric generators of the TGV-200M type. The BN-600 has a thermal power rating of 1470 MW(th) and the electrical power rating amounted 600 MW(e).
The reactor core fuel, blankets, neutron reflectors, the control and protection system including actuators, three primary coolant pumps, most of the primary coolant pipelines, six intermediate heat exchangers and associated structures and components are placed in the main reactor vessel filled with liquid sodium (primary coolant). The volume of primary sodium exceeds 800 m3. The BN-600 uses enriched uranium oxide fuel, however it was designed to generate the ‘secondary’ nuclear fuel material (plutonium isotopes) in the reactor core and blankets.
The main reactor vessel is enclosed inside the guard vessel with the gap between these two vessels chosen to keep the sodium level in the main vessel from dropping too low in the case of main reactor vessel leak. The guard vessel sits within a concrete chamber lined with a 10 mm thick steel. The top side of this chamber has a cover of an upper biological shielding.
Each secondary loop includes two intermediate heat exchangers located in the reactor vessel, a buffer tank compensating for sodium volume changes, a secondary coolant pump, pipelines and a sectional-modular steam generator. The volume of secondary sodium in every loop equals 280-300 m3.
The pressure of gas in the reactor vessel gas plenum is normally maintained at values lower than 0.2 MPa. The characteristics of the secondary circuit including the geometry of the loops have been selected in such a way that the static pressure (including gravity pressure set by the sodium level) on the secondary side of the intermediate heat exchangers exceeds the pressure on the primary side. These arrangements help to prevent potential accidental leaks of the primary sodium into the secondary circuit through the intermediate heat exchangers.
Every once-through type steam generator has an eight-section arrangement. Every section consists of the evaporation module, steam superheater module and reheater module. Each section can be disconnected from the secondary (sodium) and tertiary (steam/ water) loops when necessary.
The basic design of the BN-600 tertiary circuit is similar to fossil power plants or secondary circuits of pressurized water cooled reactors. Every loop of the tertiary circuit includes a steam/water part of the steam generator, a turbine with its auxiliary equipment, a condenser, a deaerator, three feedwater pumps with electric drives and an emergency feedwater pump.
Three independent pairs of secondary and tertiary loops in the BN-600 reactor provide for reactor cooldown during normal operation and in the case of emergency for a safety function of residual heat removal. This three-train design may create delays in the reactor maintenance processes. Hence, a special complementary cooldown system was considered for BN-600. When introduced, this system may be connected to the secondary loops through the sodium-air heat exchangers.
BN-600 operates in a base-load mode. The average load factor estimated in the reactor design documentation amounts 76% and this value corresponds to the actual performance achieved during the reactor operation once the necessary level of technology maturity had been achieved.
BN-800 reactor
The long lasting and successful operation of the BN-600 reactor preceded the design of the BN 800, which uses most of the technologies developed and mastered at the design, commissioning and operation of the BN-600. Unlike BN-600, the BN-800 reactor design objectives involved the demonstrations of BN technology competitiveness against other energy supply options and the feasibility of industrial scale implementation of the closed fuel cycle technology.
Apart from the generation of electricity and district heating the BN-800 reactor has these design objectives:
- Operation of the reactor using mixed uranium-plutonium oxide (MOX) fuel which is important part of the closed fuel cycle technology deployment;
- Preservation and continuity of knowledge, practical skills and technologies in design, construction and operation of sodium cooled fast reactors;
- Support the research development and demonstration programmes of the sodium cooled fast reactor technologies, and the development of new fuels and of the reactor core structural materials in particular;
- Testing and validation of new systems, structures and components and new computer codes.
BN-800 is an evolutionary upgrade of BN-600. The BN-800 based its normal operation systems including the sodium circuits design and sodium systems, safety systems (except new safety systems and numerous improvements), instrumentation and control systems including reactor monitoring systems on similar systems used in the BN-600. Operating and maintenance conditions, and procedures are similar to those in BN-600, and incorporate operational approaches and experience accrued over the 60 years of the national fast reactor programme.
Commissioned in 2016 (see Figure 1), the BN-800 reactor is equipped with three steam generators N-272, a turbine of the K-800-130/3000 type and an electric generator of the TZV-800-2 type. The BN-800 has a thermal power rating of 2100 MW(th) and an electrical power rating of 880 MW(e).
BN-800 is a pool type reactor using a similar layout as the BN-600 reactor described above. The volume of primary sodium in BN-800 equals 1100 m3. BN-800 can use enriched uranium oxide fuel and MOX fuel.
Like the BN-600, BN-800 uses the three circuit configuration for energy transfer and conversion. Sodium is the coolant in the primary and secondary circuits and water is the coolant in the tertiary circuit.
Each circuit consists of three identical parallel loops. Each secondary loop includes two intermediate heat exchangers located in the reactor vessel, a buffer tank compensating for sodium volume changes, a secondary coolant pump, an emergency heat removal system heat exchanger, pipelines and a sectional-modular steam generator. The volume of secondary sodium in every loop amounts approx. 350 m3. The emergency heat removal system connects to every secondary loop through the sodium-sodium heat exchanger which is connected in parallel to the steam generator.
Every steam generator consists of ten sections. Every section consists of the evaporation module and the steam superheater module. BN-800 uses steam-steam reheating scheme. Each section can be disconnected from the secondary (sodium) and tertiary (steam/water) loops when necessary.
The layout of tertiary circuit and its systems structures and components have similarity to superheated steam turbines. The tertiary circuit through the primary and secondary circuits and steam generators provides the removal of heat from the reactor at normal operation conditions, deviations from normal operation and in most accident scenarios. However, this heat removal scheme may fail in the cases of loss of feedwater, station blackout scenarios, earthquakes, etc. Unlike BN-600, the BN-800 uses a single feedwater supply system for all three steam generators which stipulates an emergency heat removal system connection in parallel to the steam generators.
The most extensive and sophisticated improvements of the BN-800 design were introduced in the systems, structures and components important to safety, and mostly incorporating new regulatory requirements. The design of BN-800 has been developed to be in compliance with the new or updated national regulatory documents on nuclear safety such as OPB-88/97, PBYa RU AS-07, SP-AS-03, etc. Classification, layout, design and construction of the NPP buildings and premises in accordance with the fire and explosion protection requirements complied with Russian fire safety standards norms documented in NPB 105-95. Nevertheless, all systems modifications and functions included the operational experience of previous installations[1].
BN-800 protective safety systems include:
- Emergency heat removal system;
- Reactor protection system;
- Reactor loss-of-coolant protection system;
- Reactor overpressure protection system;
- Secondary circuit overpressure protection system;
- Heat removal system for fuel assemblies for use during their reloading from the reactor to the spent fuel ‘drum’ storage;
- Spent fuel ‘drum’ storage heat removal system;
- Spent fuel ‘drum’ storage casing overpressure protection system;
- Guard casings of primary pressure gas pipelines and guard shell of the pressure chamber.
BN-800 confinement safety systems include:
- Reactor guard vessel;
- Reactor core catcher;
- Reactor confinement compartments and leak-tight enclosure;
- Guard casings of primary auxiliary systems pipelines;
- Primary sodium systems, structures and components compartments ventilation system and spent fuel ‘drum’ storage compartment ventilation system;
- Sodium systems, structures and components compartments fire protection system;
- Spent fuel ‘drum’ storage casing;
- Guard casings at the pipeline sections from the spent fuel ‘drum’ storage to the overflow vessel;
- Exterior lining of the spent fuel cooling pond.
In comparison with the BN-600, the modifications of the BN-800 reactor design include the following features increasing the reactor systems reliability and improving safety:
- Sealed cover was introduced around the reactor pressure chamber;
- A reactor vessel bottom part and the reactor support structure have been redesigned to improve seismic characteristics;
- The thickness walls of the reactor main vessel and guard vessel was increased from 20 to 30 mm;
- Reactor core catcher has been introduced to protect the reactor vessel from the molten fuel effects in the case of severe accident;
- Control rods with the passive actuation principles have been added to the reactor protection system;
- The primary coolant purification system has a stationary arrangement installed for separation and removal of caesium from sodium;
- System protecting the reactor from overpressure or accidental depressurization has been improved;
- New ionization chambers have been introduced to monitor the reactor core in the subcritical state;
- New reactor core design was proposed to minimize the value of sodium void reactivity effect;
- One rotating plug was added to the fuel reloading system; however, the reloading system and reloading procedures have been simplified and one in-vessel elevating machine was eliminated;
- In-vessel fuel cladding leaks detection system has been installed and interlocked with the reloading system;
- Entrainment of primary sodium from the reactor vessel to the systems and pipelines located outside of reactor vessel in the case of depressurization was ruled out;
- Fire and explosion protection of the steam generators and protection of other systems, structures and components located in the steam generator compartments have been improved;
- New emergency heat removal system has been installed and connected to the secondary circuit loops in parallel to the steam generators. The residual heat removal is provided through the independent sodium-air heat exchangers;
- Unlike the BN-600 electric generators TGV-200M that uses hydrogen for cooling, the cooling system of the BN-800 electric generator TZV-800-2 uses water as coolant;
- Filters removing radioactive aerosols from the combustion products have been added to the fire protection and ventilation systems.
BN-1200 reactor
The Russian Federation nuclear power strategy in the first half of 21 century determines tasks for the new generation nuclear power plant development and deployment[2]:
- Eliminate accidents that require public evacuation or relocation;
- Use effectively fissile and fertile materials provided by natural uranium;
- Multi-recycling of nuclear material minimizing amount of high level radioactive waste;
- Strengthening of non-proliferation characteristics of materials and technologies;
- Maintain competitiveness of nuclear power.
Minimum excess reactivity in the reactor core can be achieved through the appropriate breeding characteristics. Effective use of natural uranium resources can be achieved using a closed fuel cycle and renouncing of direct disposal of the spent fuel. Multiple recycling of nuclear materials in closed fuel cycle can help to minimize the minor actinide content in the high level waste.
The development of large sodium cooled fast reactors started in the Russian Federation a few decades ago. Design work on the BN-1200 reactor started in 2007 as part of the JSC Concern Rosenergoatom programme. Approval of the terms of reference for the development of BN-1200 reactor installation occurred in 2012. Later the development of BN-1200 moved ahead as part of the national ‘Breakthrough’ programme.
By the time of this INPRO assessment study, the basic design of the reactor installation and systems, structures and components had been developed including the design of steam generators and the core and fuel design of both MOX fuel and high density (nitride) fuel. The development of BN-1200 detailed design has been a continuous project.
The ‘Breakthrough’ Project Office and the national nuclear utility JSC Concern Rosenergoatom coordinate the BN-1200 project. The ‘Breakthrough’ Technical Committee reviews major modifications, new features and other technical issues related to the reactor development. JSC OKBM is the BN-1200 design organization responsible for the implementation of RD&D programme and design developments required. Organizations participating in the development of systems and technologies for BN-1200 have many years of experience in this area and established cooperation mechanisms, which are necessary for the assurance of design quality and safety[3][4][5][6].
The ‘new generation’ reactor designs which are being developed in the Russian Federation, including BN-1200, aim to resolve the following tasks:
- Competitiveness with other advanced nuclear power plants and with power plants using fossil fuel;
- Enhanced safety level corresponding to the requirements formulated for the Gen IV reactors, and elimination of the necessity of public evacuation/relocation in the case of potential accidents;
- Multi-recycling of plutonium isotopes in fast reactors and nuclear fissile material breeding for long term fuel supply to other types of reactors (water cooled reactors);
- Duration of the construction period up to 48 months for ‘N-th-of-a-kind’ commercial power plants;
- A feasibility of commissioning of a series of NOAK commercial power plants in 2-3 years after the FOAK power plant commissioning.
The following basic conceptual provisions have been defined as a basis for the BN-1200 design development project[7]:
- Proven technologies and experience acquired in the design, commissioning and operation of BN-600 and BN-800 reactors should have broad use in the BN-1200 design to the greatest extent possible [8][9];
- Testing and validation of improvements in the reactor safety, economic competitiveness and effectiveness of fuel management incorporating innovative technologies through the appropriate RD&D activities using existing and newly developed research facilities;
- Optimization of the infrastructure requirements can be achieved through the selection of the appropriate value of the BN-1200 electrical power rating and may involve unification of requirements for the NPP siting and unification of the electric generators and electric components used for the plant connection to the grid;
- Transportation of the NPP components to the construction site by railroad.
BN-1200 is a pool type reactor and it has an integral layout of the primary circuit like BN-800 and BN-600 where the primary circuit and radioactive primary coolant are in the main reactor vessel enclosed in the guard vessel as described above.
Like the BN-600 and BN-800, the BN-1200 reactor vessel is supported at the lower cylindrical part from the bottom side. Cooling of the reactor vessel has been worked out in a way that shielding structures provide the possibility of compact layout of the in-vessel systems, structures and components, and allows to get relatively small vessel diameter.
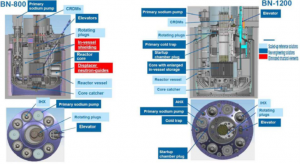
Unlike the BN-600 and BN-800, the BN-1200 reactor maintains the level of primary sodium below the tapered upper part of the main vessel. This modification eliminates the need of guard vessel and bellows in the tapered part of the reactor vessel. Use of the main reactor vessel upper tapered part for the support of reactor equipment allows accommodating the four primary coolant pumps, four intermediate heat exchangers, the emergency heat removal system heat exchangers and a cold filter trap of the primary circuit within the BN-1200 reactor vessel without increasing the vessel diameter. The latter modification eliminates components containing primary sodium outside of the reactor vessel and eliminates potential leaks of primary sodium from the reactor vessel external system. Figure 2 summarises basic similarities and distinctions between BN-1200 and BN-800 reactor vessel designs.
The four in-vessel ionization chambers improve the BN-1200 neutron flux monitoring and eliminates the need of neutron guides used in BN-600 and BN-800. A rotating roof planned for installation above the BN-1200 reactor vessel protects the reactor systems, structures and components from the potential falling of heavy objects.
Like BN-600 and BN-800, the BN-1200 uses the three-circuit configuration for the energy transfer and conversion. Sodium is used as a coolant in primary and secondary circuits, and water in tertiary circuit. However, in the BN-1200 every circuit contains four loops.
Each secondary loop is physically separated from three other secondary loops and located in a separate compartment. The loop includes a single intermediate heat exchanger located in the reactor vessel, a secondary coolant pump, pipelines and a sectional-modular steam generator. In BN-1200 the guard casings is introduced in the most of secondary pipelines. The buffer tanks compensating for the secondary sodium volume changes is combined with the tanks of secondary coolant pumps.
In order to reduce the length of pipelines in the secondary circuit and to minimize the number of installed isolating valves the bellows, compensators have been introduced in the BN-1200 reactor. Further reducing the volume of building and materials used the shell-and-tube type steam generators have been introduced in BN-1200 instead of sectional-modular type steam generators in BN-600 and BN-800 (see Table 1 and Figure 3).
Specific volume | BN-800 | BN-1200 |
---|---|---|
Main building, m3/MW(e) | 750 | 525 |
Steam generators boxes, m3/MW(e) | 32 | 16 |
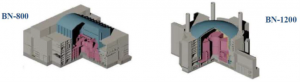
The tertiary circuit layout of BN-1200 includes the K-1200-16.0/50 turbine and steam-steam reheating scheme. To increase the plant thermal efficiency to 43.6% (gross), the temperature and pressure of live steam, and feed water have been increased compared to the BN-800 parameters. BN-1200 uses electric generator of the type which is used in other advanced water cooled NPPs of that power rating designed in the Russian Federation (AES-2006).
In comparison with BN-600 and BN-800, other than the discussed above modifications of BN 1200 reactor design for increasing the reactor systems reliability and improving safety include the following[11][12]:
- Use of uranium-plutonium nitride fuel helps to reduce the excess reactivity at full power conditions mitigating the potential consequences of RIA type of accidents. The BN-1200 excess reactivity at full power conditions will not exceed 0.5% Δk/k;
- Simultaneous withdrawal of more than one control rods from the reactor is prevented by multiple independent measures;
- Passive high temperature actuated control rods system is introduced in BN-1200 in addition to the passive hydraulically suspended control rods system20 and active shut down system;
- Maximum power density in the reactor core is reduced to 380 MW/m3;
- Duration of spent fuel storage within the reactor vessel is extended to two years reducing the spent fuel assembly power density to 2 W/cm3, simplifying the fuel reloading process and improving safety of spent fuel management;
- Emergency heat removal system is based on the passive removal of heat from the reactor to the environment through the sodium-sodium and sodium-air heat exchangers, and designed in such a way that in the case of emergency cooling the primary sodium circulates through the reactor core fuel assemblies driven by natural circulation flow;
- The above the reactor premise is used for confinement of radioactive gases and aerosols prior to subsequent filtration in the special ventilation system reducing the radioactivity of released gases. Calculated core damage frequency of the BN-1200 reactor (approx. 5×10-7 1/a) is essentially reduced compared with those of BN-800 (approx. 2×10-6 1/a) and BN-600 (approx. 10-5 1/a).
INPRO sustainability assessment of BN-1200 in the area of economics
This section presents an overview of the assessment method used in the INPRO area of economics and modifications to the method that have been introduced in the study. It provides input parameters used in the calculations and basic assumptions made by the national experts performing such calculations. Finally, the results of calculations presented in this section may be considered as an input for further research activities focused on optimization of the fast reactors designs and fuel cycles, however they should not be considered in relation to any commercial activities.
Overview of the application of the INPRO methodology area of economics to the fast reactors under development
The INPRO methodology has been developed for the sustainability assessment of the nuclear energy systems comprising different types of reactors and fuel cycle facilities. Sustainability assessment using INPRO methodology covers several different areas including economics, reactor safety, safety of fuel cycle facilities, waste management, etc. However, in the area of economics the INPRO methodology focuses on economic characteristics of energy products, i.e. electricity, heat, etc, generated by an NPP, rather than on economic parameters of fuel cycle facilities which are used as an input in a form of ‘cost of a given service’. For example, unless such calculations are the only way of obtaining costs of services the assessor is not required to calculate the figures of merit of the enrichment or reprocessing facility for the purpose of INPRO sustainability assessment. Instead, the costs of enrichment or reprocessing services are aggregated along with other costs in the figures of merit of the NPP producing final energy product. These figures of merit are used for the assessment against INPRO criteria in the area of economics.
INPRO methodology recommendations have the same hierarchy in all areas of assessment. On top of this structure a basic principle of sustainability is defined for every area determining a goal to be achieved to make the nuclear energy system sustainable[13]. The necessary actions to be taken to achieve the goal defined in the basic principle are introduced on the second level and called ‘user requirements’. At the next and third level, every user requirement splits into a few criteria. Criteria are the tools for assessment. Every criterion consists of an indicator (e.g. parameter) and an acceptance limit defining the range of values satisfying this criterion.
The INPRO assessment is normally done on the criteria level. When all criteria comprising a given user requirement are met, it means that necessary action occurred in a correct manner to meet the user requirement. When all given user requirements in a given area are met, the goal defined in the corresponding INPRO basic principle is also met[13]. However, the INPRO methodology assessment is not only used for the confirmation of nuclear energy system sustainability. The INPRO assessment criteria which are not met provide valuable information on the gaps in the nuclear power programme and help to define follow-up actions (e.g. R&D) necessary to close these gaps. Comparison of the different assessment results may help to estimate the potential advantages of the assessed nuclear energy systems.
Judgement on the system sustainability can be derived from the results of full scope INPRO assessment in all INPRO methodology areas. However, preliminary results of the assessment in selected areas can be used for the optimization of nuclear energy system or modification of the selected system components. Although such limited scope assessments can provide valuable information on the system sustainability, the judgement on sustainability can be concluded only in the full scope INPRO sustainability assessment study in all areas.
This limited scope assessment study omits INPRO areas involving the essential numbers of country specific criteria (waste management, infrastructure, proliferation resistance) and focuses on the two areas encompassing the most of design related information – economics and reactor safety.
The INPRO methodology in the area of economics sets up the goal of achieving ‘affordable and available’ produced energy and related products and services in a sustainable nuclear energy system[13]. The affordability of energy is understood as cost competitiveness with alternatives available in the country or region. The availability of nuclear energy is seen as the ability to finance the project at acceptably low investment risk.
Four user requirements have been introduced in the INPRO methodology area of economics to specify how the basic principle can be met[13]:
- The cost of energy supplied by nuclear energy systems, taking all relevant costs and credits into account, should be competitive with that of alternative energy sources, that are available for a given application in the same time frame and geographic region/jurisdiction;
- The total investment required to design, construct, and commission nuclear energy systems, including interest during construction, should be such that the necessary investment funds can be raised;
- The risk of investment in nuclear energy systems should be acceptable to investors;
- Innovative nuclear energy systems should be compatible with meeting the requirements of different markets.
The developer of sustainable energy technology is expected to meet these user requirements. The INPRO assessor has eight criteria which can be used for checking the status of nuclear energy system in relation to the INPRO user requirements:
- Cost competitiveness;
- Attractiveness of investment;
- Investment limit;
- Maturity of design;
- Construction schedule;
- Uncertainty of economic input parameters;
- Political environment;
- Flexibility.
Only five of these eight criteria have been assessed in this study as explained below. The cost competitiveness criterion uses the cost of energy generated by the nuclear energy system as an indicator, i.e. characteristic to be assessed. The limit for acceptance of this characteristic was defined as:
INPRO Assessment of the Planned Nuclear Energy System of Belarus
References
- ↑ 1.0 1.1 1.2 1.3 BAKLUSHIN R., Technology of sodium cooled NPP. History of the development and operating experience, (in Russian), SSC RF-IPPE Publ., Obninsk, Russian Federation (2013).
- ↑ GOVERNMENT OF THE RUSSIAN FEDERATION, Russian nuclear energy development strategy in the first half of the XXI century, Minutes No.17A (in Russian), Moscow (2000).
- ↑ SHEPELEV, S., BN-1200 Design (in Russian), Conference on ‘New technology platform of nuclear power: ‘Break-through’ project’, Rosatom, Moscow (2014).
- ↑ VASIL’EV, V., et al, Improvement of the Equipment in Fast-Neutron Reactor Facilities, Atomic Energy, Vol. 108, No. 4, Moscow (2010)
- ↑ АSHIRMETOV, M., VASIL'EV, B., POPLAVSKIJ, V., SHEPELEV, S., BN-1200 Design Development, Proceedings of Int. conf. FR-13, Paris (2013)
- ↑ АSHIRMETOV, M., VASIL'EV, B., POPLAVSKIJ, V., SHEPELEV, S., Design of Beloyarsk NPP power unit 5 with BN-1200, (in Russian), Proceedings of 9th Int. scientific technical conf. ‘Beloyarsk NPP - 50 years’, Zarechnyj, Russian Federation (2014).
- ↑ RACHKOV, V., et al, Concept of an Advanced Power-Generating Unit with a BN-1200 Sodium-Cooled Fast Reactor, Atomic Energy, Vol. 108, No. 4, Moscow (2010).
- ↑ OSHKANOV, N., et al, Thirty Years of Experience in Operating the BN-600 Sodium Cooled Fast Reactor, Atomic Energy, Vol. 108, No. 4, Moscow (2010).
- ↑ SARAEV, O., et al, BN-800 Design Substantiation and Status of Construction, (in Russian), Atomic Energy, Vol.108, No.4 (2010).
- ↑ 10.0 10.1 INTERNATIONAL ATOMIC ENERGY AGENCY, Fast Reactors and Related Fuel Cycles: Next Generation Nuclear Systems for Sustainable Development (FR17), Proceedings of an international conference, IAEA Proceedings Series, IAEA, Vienna (2018).
- ↑ VASIL'EV, B., et al, Implementation of the Principle of Inherent Safety in the BN-1200 Design, (in Russian), Safety of nuclear technologies and the environment, No.1, Moscow (2012).
- ↑ KUZNETSOV, I., POPLAVSKIJ, V., Safety of NPP with fast reactors, (in Russian), IzdАt Publ., Moscow (2012).
- ↑ 13.0 13.1 13.2 13.3 INTERNATIONAL ATOMIC ENERGY AGENCY, INPRO Methodology for Sustainability Assessment of Nuclear Energy Systems: Economics, IAEA Nuclear Energy Series No. NG-T-4.4, IAEA, Vienna (2014).