Waste Management (Sustainability Assessment)
INPRO basic principle (BP) for sustainability assessment in the area of waste management – Radioactive waste in a NES is managed in such a way that it will not impose undue burdens on future generations.
Contents
- 1 Introduction
- 2 General features of waste management
- 2.1 The concept of sustainable development and its relationship to the INPRO methodology in the area of waste management
- 2.2 NES activities producing waste
- 2.3 Overview of waste management
- 2.4 Classification and categorization of radioactive waste
- 2.5 End states of nuclear waste
- 2.6 Organisations for waste management
- 2.7 Cost of waste management
- 3 Necessary INPUT for a sustainability assessment in the area of waste management
- 4 INPRO basic principle, user requirements and criteria for sustainability assessment in the area of waste management
- 4.1 Relationship of the safety fundamentals and nuclear energy principles to the INPRO methodology
- 4.2 INPRO Basic principle for sustainability assessment in the area of waste management
- 4.3 User requirement UR1: Classification, categorization and minimization of waste
- 4.4 User requirement UR2: Predisposal waste management
- 4.5 User requirement UR3 End state
- 5 Appendix I
- 6 Appendix II
- 7 References
Introduction
Objective
This volume of the updated INPRO manual provides guidance to the assessor of a planned NES (or a facility) on how to apply the INPRO methodology for sustainability assessment in the area of waste management. The INPRO assessment is expected either to confirm the fulfilment of all INPRO methodology waste management criteria or to identify which criteria are not fulfilled and note the corrective actions (including research, development and demonstration) that would be necessary to fulfil them.
This publication is intended for use by organizations involved in the development and deployment of a NES including planning, design, modification, technical support and operation for waste management facilities. The INPRO assessor (or a team of assessors) is assumed to be knowledgeable in the area of radioactive waste management and/or may be using the support of qualified national or international organizations (e.g. the IAEA) with relevant experience and expertise. It is not necessary, however, for the assessor to be a specialist in waste management. The assessor should, nevertheless, be sufficiently familiar with the subject area to be able to judge whether or not a given INPRO methodology criterion has been satisfied. The manual has been written to meet the requirements of such an assessor. In some sections, this may lead to simplifications and wording that may differ from the wording used in the IAEA Safety Standards.
Scope
This manual provides guidance for assessing the sustainability of a NES in the area of waste management. Waste generated by NESs and considered in this publication includes all classes and categories of waste from nuclear power plants and nuclear fuel cycle facilities over the course of normal operations and anticipated operational occurrences. It is recognized that a given Member State may adopt additional criteria with indicators and acceptance limits that are more relevant to its circumstances. Accordingly, the information presented in Chapter 4 (INPRO methodology criteria, user requirements and basic principle for sustainability assessment of NESs in the area of waste management) can be viewed as guidance.
This INPRO methodology manual does not establish any specific safety requirements, recommendations or criteria. The INPRO methodology is an internationally developed metric for measuring nuclear energy system sustainability and is intended for use in support of nuclear energy system planning studies. IAEA safety requirements and guidance are only issued in the IAEA Safety Standards Series. Therefore, the basic principles, user requirements and associated criteria contained in the INPRO methodology should only be used for sustainability assessments. The INPRO methodology is typically used by Member States in conducting a self-assessment of the sustainability and sustainable development of nuclear energy systems. This INPRO methodology manual should not be used for formal or authoritative safety assessments or safety analyses to address compliance with the IAEA Safety Standards or for any national regulatory purpose associated with the licensing or certification of nuclear facilities, technologies or activities.
The manual does not provide guidance on implementing waste management activities in a country. Rather, the intention is to check whether such activities and processes are (or will be) implemented in a manner that satisfies the INPRO methodology criteria, and hence the INPRO user requirements and basic principle for sustainability assessment in the area of waste management.
This manual does not specifically consider the emissions of environmental stressors from waste management facilities but does include a generic discussion of safety cases for predisposal waste management facilities and end states. Radioactive and other emissions (chemicals, heat, etc) arising during normal operations and anticipated deviations from normal operations of NES facilities, including unconditional and conditional releases of waste, are discussed in a separate manual for the INPRO area of environmental impact of stressors[1]. Safety-related sustainability considerations for waste management installations operating as part of NPPs or nuclear fuel cycle facilities, including spent fuel storage and reprocessing facilities, are covered in the manuals for the INPRO areas of safety of reactors and fuel cycle facilities, i.e. in Volumes 8 and 9 of Ref[2]. Radioactive and chemical emissions during accidents are likewise covered in the manuals for the INPRO areas of safety of reactors and fuel cycle facilities.
Specific issues for managing radioactive wastes resulting from accidents or from military activities, research facilities, medical uses, and agricultural applications are beyond the scope of this publication. However, selected INPRO criteria might also be found applicable and useful for the planning of waste management in these areas.
Structure
This publication follows the relationship between the concept of sustainable development and different INPRO methodology areas. Section 2 describes the linkage between the United Nations Brundtland Commission’s concept of sustainable development and the IAEA’s INPRO methodology for assessing the sustainability of planned and evolving NESs in the area of waste management. It considers general features of waste management and presents relevant background information. Section 3 identifies the information that needs to be assembled to perform an INPRO assessment in the area of waste management. Section 4 provides guidance on assessing compliance with the INPRO waste management criteria and, hence, the user requirements and the basic principle for sustainability assessment in the area of waste management. Appendix I briefly describes the concept of ALARP, i.e. as low as reasonably practicable, taking social and economic factors into account. Appendix II presents normalized waste arisings of different NESs with different types of reactors based on average values of historical data.
Table 1 provides an overview of the INPRO basic principle (BP), user requirements (UR) and criteria (CR) for sustainability assessment in the area of waste management.
INPRO basic principle for sustainability assessment in the area of waste management: Radioactive waste in a nuclear energy system is managed in such a way that it will not impose undue burdens on future generations. | ||
INPRO user requirements | Criteria | Indicator (IN) and Acceptance Limit (AL) |
---|---|---|
UR1: Classification, categorization, and minimization of waste.
The radioactive waste is classified and categorized to facilitate waste management in all parts of the NES, and the NES is designed and operated to minimize the generation of waste at all stages, with emphasis on waste containing long-lived radio-toxic components that would be mobile in a repository environment. |
CR1.1: Waste classification and categorization | IN1.1: Classification and categorization scheme. |
AL1.1: The scheme permits unambiguous, practical segregation for processing, storage and disposal, and identification of waste arisings. | ||
CR1.2: Waste minimization | IN1.2: Characteristics of waste generated by the NES. | |
AL1.2: NES waste specific characteristics (mass, volume, total activity; amount of alfa-emitters, long-lived radionuclides and chemically toxic elements) have been minimized. | ||
UR2: Predisposal waste management. Intermediate steps between generation of the waste and the end state are taken as early as reasonably practicable. The processes do not inhibit or complicate the achievement of the end state.
|
CR2.1: Process descriptions | IN2.1: Process descriptions that encompass the entire waste life cycle. |
AL2.1: The complete chain of processes from generation to final end state is described in sufficient detail to make evident the feasibility of all steps. | ||
CR2.2: Time for waste form production | IN2.2: Time to produce the waste form specified for the end state. | |
AL2.2: Consistent with the schedule for transfer of the waste to its end state. | ||
CR2.3: Predisposal waste management safety | IN2.3: Safety case for predisposal waste management facilities. | |
AL2.3: Meets national regulatory standards and is consistent with applicable international safety standards. | ||
UR3: End state. An achievable end state that provides permanent safety without further modification is specified for each class of waste. The waste is brought to this end state as soon as reasonably practicable.
|
CR3.1: End state technology | IN3.4: Availability of end state technology. |
AL3.4: End states are identified for all waste streams and all required technology is currently available or reasonably expected to be available on a schedule compatible with the schedule for introducing the waste management for all NES facilities. | ||
CR3.2: Safety of end state | IN3.2: Safety case for the end state. | |
AL3.2: Meets national regulatory standards and is consistent with applicable international safety standards. | ||
CR3.3: Schedule for achieving end state | IN3.3: Time to reach the end state. | |
AL3.3: As short as reasonably practicable. | ||
CR3.4: Resources for achieving end state | IN3.4: Availability of resources. | |
AL3.4: Resources (funding, space, capacity, etc.) are available for achieving the end state, compatible with the size and growth rate of the NES. Costs of all waste management steps are included as a specific line item in the product’s cost estimate. |
General features of waste management
This section provides general background information on management of radioactive waste arising in a NES. Radioactive waste is inevitably produced during nuclear power operations or nuclear materials applications in research, industry or medicine. The generated waste will need to be managed safely. The INPRO methodology is focused on the sustainability of NESs. Accordingly, this manual address only the assessment of sustainability related issues involving the management of waste generated by NES facilities, meaning nuclear power production and nuclear fuel cycle facilities.
The concept of sustainable development and its relationship to the INPRO methodology in the area of waste management
The United Nations World Commission on Environment and Development Report[3] (often called the Brundtland Commission Report), defines sustainable development as “development that meets the needs of the present without compromising the ability of future generations to meet their own needs” (para.1). This definition:
“contains within it two key concepts:
- the concept of ‘needs’, in particular the essential needs of the world’s poor, to which overriding priority should be given; and
- the idea of limitations imposed by the state of technology and social organization on the environment’s ability to meet present and future needs.”
Based on this definition of sustainable development, a three-part test of any approach to sustainability and sustainable development was proposed within the INPRO project: 1) current development should be fit to the purpose of meeting current needs with minimized environmental impacts and acceptable economics, 2) current research, development and demonstration programmes should establish and maintain trends that lead to technological and institutional developments that serve as a platform for future generations to meet their needs, and 3) the approach to meeting current needs should not compromise the ability of future generations to meet their needs.
The definition of sustainable development may appear obvious, yet passing the three-part test is not always straightforward when considering the complexities of implemented nuclear energy systems and their many supporting institutions. Indeed, many approaches may only pass one or perhaps two parts of the test in a given area and fail the others.
The Brundtland Commission Report’s overview (para. 61[3]) on nuclear energy summarized the topic as follows:
“After almost four decades of immense technological effort, nuclear energy has become widely used. During this period, however, the nature of its costs, risks, and benefits have become more evident and the subject of sharp controversy. Different countries world-wide take up different positions on the use of nuclear energy. The discussion in the Commission also reflected these different views and positions. Yet all agreed that the generation of nuclear power is only justifiable if there are solid solutions to the unsolved problems to which it gives rise. The highest priority should be accorded to research and development on environmentally sound and ecologically viable alternatives, as well as on means of increasing the safety of nuclear energy.”
The Brundtland Commission Report presented its comments on nuclear energy in Chapter 7, Section III[3]. In the area of nuclear energy, the focus of sustainability and sustainable development is on solving certain well known problems (referred to here as ‘key issues’) of institutional and technological significance. Sustainable development implies progress and solutions in the key issue areas. Seven key issues are discussed in Ref[3] (in this order):
- Proliferation risks;
- Economics;
- Health and environmental risks;
- Nuclear accident risks;
- Radioactive waste disposal;
- Sufficiency of national and international institutions (with particular emphasis on intergenerational and transnational responsibilities);
- Public acceptability.
The INPRO methodology for the self-assessment of sustainability and sustainable development of a NES is based on the broad philosophical outlines of the Bruntland Commission’s concept of sustainable development described above. Although three decades have passed since the publication of the Brundtland Commission Report and eighteen years have passed since the initial consultancies on development of the INPRO methodology in 2001 the definitions and concepts remain valid. The key issues for sustainable development of NESs have remained essentially unchanged over the intervening decades, although significant historical events have starkly highlighted some of them.
During this period, several notable events have had a direct bearing on nuclear energy sustainability with regard to non-proliferation, nuclear security, waste management, cost escalation of new construction and, most notably. safety.
Each INPRO methodology manual examines a key issue of NES sustainable development. The structure of the methodology is a hierarchy of INPRO basic principles, INPRO user requirements for each basic principle, and specific INPRO criteria measuring whether each user requirement has been met. Under each INPRO basic principle for the sustainability assessment of NESs, the criteria include measures that take into consideration the three-part test based on the Brundtland Commission’s definition of sustainable development as described above.
This INPRO manual focusses on the key issue of radioactive waste management associated with NES development and deployment. This manual does not specifically consider emissions of environmental stressors from waste management facilities except in the generic discussion of safety cases for predisposal waste management facilities and end states. The radioactive and other (chemicals, heat, etc.) emissions during normal operation and deviations from normal operation of the NES facilities, including unconditional and conditional releases of waste, are discussed under the INPRO area of environmental impact of stressors and published in a separate manual[1]. Safety issues of waste management installations operating as part of NPP or nuclear fuel cycle facilities, and safety of spent fuel storage and reprocessing facilities are covered under the INPRO areas of safety of reactors and fuel cycle facilities published in Volumes 8 and 9 of Ref[2]. The radioactive and chemical emissions during accidents are also covered under the INPRO areas of safety of reactors and fuel cycle facilities.
Historically, national nuclear power programmes have failed to address several important waste management aspects in a sufficient and timely manner, and those aspects have increasingly become hot topics in the area of public acceptance. Countries with nuclear power or nuclear fuel cycle facilities within their territory need to demonstrate real progress on implementing waste management practices. Countries planning to embark on a nuclear power programme have to plan commensurate waste management activities in parallel. Waste management expertise needs to be built up in parallel with developing the capacity to operate an NPP. Wastes need to be managed safely on an interim basis before final disposal. Plans need to be in place to establish disposal facilities, in due course, and progress needs to be made in moving towards disposal. Expertise is needed for both managing and planning and this usually means that a dedicated waste management organization has to be established.
Nuclear power technologies produce radioactive waste that needs to be managed safely. Being in the nuclear business therefore means being in the radioactive waste management business. The extent of a country’s waste management business is commensurate with the extent of its nuclear business. A country’s operating nuclear power plants need to have a reasonably well developed waste management system within that country. Some waste management facilities may be incorporated into the design of the NPP, such as on-site facilities for interim storage of spent fuel and operational wastes, and these may come into operation at the same time as the plant. Other facilities will need to be established in due course.
Today, there are discussions about possible regional waste management disposal facilities. However, because such facilities do not yet exist, it is recommended that countries achieve sustainability by basing their plans on having to dispose of radioactive wastes within their own territory unless other arrangements are envisioned through special bilateral or multilateral agreements (see e.g. Refs[4][5]).
Planning for, and analysing what is needed to develop, a robust safety case for disposal can avoid pitfalls in interim steps and resulting needs for remedial activities. Planning for a nuclear power plant means also planning for managing the waste from the power plant, including not only short term storage but all steps through to final disposal.
The general goal of waste management is to manage radioactive wastes so as to protect human health and the environment, from the time that the waste is produced and into the future, with no limit over time or space. Consideration needs to be given to how, when and where harm might arise and to measures necessary to prevent it. The general goal also includes managing wastes in a manner that minimizes the burden passed to future generations. The generation that enjoys the benefits of the activities that give rise to the waste should assume responsibility for it and, to the extent possible, not pass this responsibility to their children and grandchildren. It can be said that we should operate nuclear energy systems in accordance with the ‘user-pays principle’.
For a complete nuclear energy system and even for a single NPP, there are potentially many different waste streams. Operational wastes in an NPP may arise from the active laundry, from decontamination and maintenance of equipment such as valves, from the primary heat transport system and its clean-up system, from the storage pool and its clean up system, etc. There are many potential steps in waste management, depending on the waste. For example, some liquid wastes may be treated to remove most of the contamination, so that the treated liquid may be released to the environment and contamination removed from the liquid will represent a new waste that has to be managed, e.g. by stabilizing it in concrete and then placing it into storage. Waste storage or spent fuel storage are the interim steps. Eventually, all wastes have to be placed in an end state with the intention of not treating them further. Ideally, the safety of an end state has to be passive, i.e. does not require human intervention and does not depend on energy supply. A waste management plan is required for each waste stream of each facility of a nuclear energy system. The plan sets out how the waste is to be processed and managed from its generation through to its placement in a safe end state. There are many potential waste streams and so there are, in principle, many individual plans. Waste management activities also produce wastes that need to be managed.
End states may include free or unconditional release, e.g. release of liquids after they have been monitored to confirm that they meet release criteria agreed with the national regulatory body, monitored gas releases through a stack after filtering, and release of decontaminated metals or other materials, again after monitoring to confirm that free release criteria are met. End states may also include recycling and/or reuse of materials, tools, equipment, etc., although in due course such material may appear again as waste, e.g. when the plant is decommissioned. Monitored release and reuse are often part of on-going operations and can be often not thought of as a part of waste management.
End states also include placing the waste in an engineered and licensed disposal facility, where the waste can be safely confined for as long as it represents a hazard and for which a safety case has been prepared to demonstrate the long term protection of human health and the environment. Depending on the class of waste, the disposal facilities may include surface mounds and subsurface disposal, near surface engineered systems and shallow rock caverns, and geological disposal. More details of the end state options are discussed in Section 2.5.
In the past, perpetual storage has also been discussed as a potential end state in which long lived wastes would be moved from one storage facility to another, as the storage facility reached its end of life, in perpetuity. Theoretically speaking, this approach could be found economically attractive under certain conditions for some classes of waste, e.g. HLW and SNF, bearing in mind the essential difference in the specific cost between the storage and disposal facility and relatively high discount rates used in many countries for such projects. Occasionally, in some countries, the question of perpetual storage can be raised as an option, e.g. in interactions with the public. However, the INPRO methodology considers geological disposal to be superior, principally because the burden passed to future generations is much lower.
For disposal of waste in an engineered facility, a safety case has to be developed to demonstrate its safety now and in the future. For a disposal facility, safety has to be demonstrated over long time frames following waste emplacement, and not just during the operation stage of this facility, i.e. the safety case for disposal of waste is fundamentally different from that for an operating nuclear facility.
To minimize the burden passed to future generations, disposal facilities, ideally, need to be passively safe in the long term. That means they will not require energy supply and human oversight or intervention. Once the waste is placed in a disposal facility, it can be left there indefinitely with no need for monitoring or any other action. Passive safety, however, does not mean that oversight cannot be maintained if society wants to do so, as long as the oversight activities, including any associated monitoring, do not impair the passive safety. Further, passive safety does not mean that waste is not potentially retrievable for a future society. This is an important point for countries that consider adopting a once through fuel cycle.
Disposing of spent fuel in an engineered geological disposal facility may not necessarily mean that the spent fuel cannot be retrieved. It means that there will not be a need to take any further action from the point of view of safety and environmental protection. But if a future society decides to retrieve the waste for some reason, it could do so, e.g. to reprocess it. By implementing disposal of spent fuel, such countries demonstrate that there is a waste management solution for long lived highly active wastes such as spent fuel and, eventually, for the high-level waste from reprocessing. Providing for the waste to be emplaced in a retrievable manner enhances the possibility of reversing decisions in repository development and provides an additional degree of flexibility, such as allowing the on-going development of the repository to respond to new technical information or policy directives.
The declared and demonstrated possibility of retrieving the waste at each stage after emplacement may also have public and political confidence benefits, in that it removes the concerns that some decision-makers may have about committing irreversibly to a given decision. There may, however, be technical, policy-related, and security disadvantages which deserve consideration. In particular, the application of nuclear safeguards to a repository in which the wastes remain ‘retrievable’ has not been worked out yet and deserves further attention. In addition, there is an argument that ‘retrievability’ runs counter to the primary objectives of geological disposal to provide permanent safety and not to facilitate irresponsible attempts to retrieve the waste or repository materials. The present consensus amongst the engaged technical community is that ‘retrievability’ can be considered in geological disposal programmes, but that it is not essential for safety[6]. If incorporated, it can be considered consistent with the primary objective of providing adequate long-term safety and security only if it is implemented in such a way as not to reduce long-term passive safety, to preserve adequate security, and not to impose undue burdens on future generations.
The INPRO methodology in the area of waste management is not guidance on how to implement waste management in a given nuclear energy system. This manual provides a tool for assessing whether the waste management is being done well enough to achieve a sustainable nuclear energy system. To judge the sustainability of the NES, all components from the front end facilities to the back end facilities need to be included in the INPRO assessment. The complete fuel cycle has many different types of waste. A full scope INPRO assessment in the area of waste management is expected to consider all such wastes. However, where a country is considering its first NPP, it may be better to start with a focus on just a few wastes – for example operational waste and spent fuel from the NPP – and then extend the thinking and assessment to include all wastes.
NES activities producing waste
Generally, an INPRO assessment is carried out for a specific NES that has been defined in an energy system planning study and meets the energy demand over time of a specific energy scenario in a country (see overview manual of the INPRO methodology[2]). For assessing compliance with the INPRO methodology basic principle and user requirements in the area of waste management, the assessment needs to take into account the complete NES, i.e. all its components (facilities), so that an adequate estimate of waste arising from the entire system, including those from decommissioning of the NES components, can be obtained. The waste arising from a number of NES activities needs to be taken into account in the assessment, including those from:
- Mining and milling;
- Uranium refining, conversion and enrichment;
- Fuel fabrication;
- Reactor operation;
- Fuel reprocessing;
- Waste processing;
- Decommissioning; and
- Waste transportation, and storage.
For the majority of these NES activities, there already exists extensive experience with managing the associated nuclear wastes, including their final disposition. This experience should be referenced in performing any assessment of a NES and especially for a country that is embarking on a nuclear power program. Given this experience base, the emphasis of an INPRO assessment in a country with an operating nuclear power programme may, in many cases, be focused on NES components (facilities) that represent a significant departure from past experience, i.e. an innovation.
Overview of waste management
In general, the management of waste involves a number of steps, as illustrated schematically in Fig. 1, leading to final disposition of the waste, namely placing it into its end state. The end state needs to be such that, ideally, long-term safety is assured without the need for institutional control. This does not mean that, once waste has been placed into such a passively safe end state, society would not seek to maintain institutional control, but rather that safety would be assured even if such controls were not maintained in the long term.
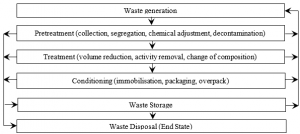
As can be seen from Fig. 1, a number of intermediate steps may be taken prior to placing a waste in its end state. Examples of intermediate steps are: segregating the waste in accordance with available processing options, treating the waste to reduce volume and increase concentration per mass, conditioning the waste to ensure that it will be chemically stable in its end state (one factor contributing to passive safety), or processing gaseous or liquid wastes to remove the radioactive components so that the purified liquid or gas can be discharged or managed as a non-radioactive material. For a given waste stream or type of waste, a management scheme will have been (or will need to be) specified so that it is safely managed through a variety of intermediate steps leading to its end state. For the most part, passively safe end states are achieved using a process of concentration and confinement.
However, for some wastes, particularly gaseous and liquid wastes, the end state may be a controlled release to the environment where the wastes are dispersed and diluted. But, for both the concentrate and confine approach and the dilute and disperse approach, the intermediate steps, i.e. waste treatment, conditioning and packaging, have to be defined taking into account the expected end state. At the same time, the intermediate steps have to be consistent with ensuring that the waste is safely managed until the end state is achieved and, in the case of storage, represents an interim method of safely isolating the waste until it is placed in its end state.
For a given waste, the classification and categorization of the waste and knowledge of its properties (radiological, physical, chemical, origin, etc.) will lead to a management strategy that sets out the various intermediate steps and the end state.
Classification and categorization of radioactive waste
Classification of waste
Although terms such as low level, intermediate level, etc are used to classify waste, it is the properties of the waste streams, waste forms, disposal packages, and end state facility designs that ultimately decide what wastes end up in what kinds of disposal facilities. Substantial work is required to classify and categorize the different waste streams that may arise in a given nuclear facility and on-going effort is necessary to ensure that the steps in processing and storage do not adversely impact the safety case for a given end state.
Nonetheless, the use of a broad classification scheme is helpful for communication among workers, organizations and nations when discussing waste management plans. The concept of a waste classification system allows identification of waste with sufficiently low activity concentrations that it could be disposed of in near-surface disposal facilities or the waste that needs to be disposed of in geological disposal facilities with more robust containment and isolation features. In addition, classification is a good tool to allow identification of waste that can be cleared from control with regard to radiation safety.
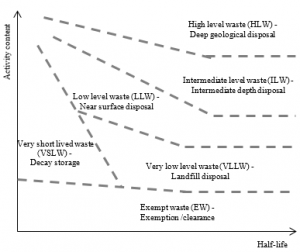
Waste classes[8] based on radioactivity concentrations and species content to be used to determine the corresponding mode of disposal include the following:
- Exempt Waste (EW) has such a low concentration of radioactive nuclides that it can be cleared from further regulatory control in accordance with the criteria to be defined by the regulator[9], as it does not require provisions for radiation protection. There are no radiological restrictions for disposal in conventional landfills or recycling;
- Very Short Lived Waste (VSLW) is waste containing primarily radionuclides that decay to insignificant levels within a period of up to a few years, and that can be stored for decay at the nuclear facility and subsequently cleared from regulatory control for uncontrolled disposal, i.e. become exempt waste;
- Very Low Level Waste (VLLW) does not comply with clearance criteria of exempt waste and can contain radionuclide levels one to two orders of magnitude above these criteria but does not need a high level of containment and isolation and, therefore, is suitable for disposal in engineered near surface landfill type facilities with limited regulatory control. Examples of VLLW are wastes from operation and decommissioning of nuclear facilities[10][11][12] with levels of activity slightly above the clearance level or residuals from mining and milling;
- Low Level Waste (LLW) exceeds exemption status but contains primarily short lived radionuclides (half-life < 30 years) with limited amounts of long lived radionuclides (half-life > 30 years). Some countries[13][14][15] limit the amount of long-lived alpha emitters (e.g. 239Pu, 241Am) to 4000 Bq/g in individual waste packages (or to an overall average of 400 Bq/g), but for long-lived gamma and beta emitting radionuclides (e.g. 14C, 36Cl, 63Ni, 93Zr, 94Nb, 99Tc and 129I) the allowable activity may be up to tens of kBq/g. LLW covers a wide range of activity concentrations: It can have an activity level just above that for VLLW not requiring shielding, isolation and containment, up to levels of activity concentration that requires shielding, robust isolation and containment for periods up a few hundred years. LLW is generally suitable for disposal in engineered near surface disposal facilities;
- Intermediate Level Waste (ILW) contains amounts of long-lived (half-life > 30 years) radionuclides making it unsuitable for engineered near surface disposal. Disposal in facilities at greater depth than near surface facilities, i.e. in the order of tens of meters to a few hundred meters is necessary for ILW. However, ILW needs no provision, or only limited provision, for heat dissipation by radioactive decay during its storage and disposal;
- High-Level Waste (HLW) is described as waste with long-lived radionuclide concentrations exceeding the limitations for short-lived Low and Intermediate Level Waste (LILW). HLW requires a higher degree of isolation from the environment for long periods of time. HLW typically has levels of activity concentration in the range of 104 to 106 TBq/m3 (e.g. for spent fuel directly after removal from power reactor core, which some States consider radioactive waste). HLW includes conditioned waste arising from the reprocessing[16] of spent fuel together with any other waste requiring a comparable degree of containment and isolation. At the time of disposal, following a few decades of cooling time, waste containing such mixed fission products typically has levels of activity concentration of around 104 TBq/m3, and a significant heat output, by the radioactive decay. These characteristics need to be considered in the disposal facility for such waste, which needs to be located in deep, stable geological formations several hundred meters or more below the surface, considering surrounding geothermal conditions and barrier performance of the facility.
Fig. 2 illustrates for each class of waste presented above its potential end state (disposal facility), based on activity content and half-life of the radioactive nuclides in the waste[8].
Categorization of waste
Classifying wastes based solely on radioactivity concentrations and species content is plausible, if it is based on the long-term safety aspects of waste disposal; however it has been proven that this approach needs to be supplemented or differentiated further by categorization of waste[8][17][18] so as to include such factors as origin, type of waste, properties, and process options to be viable for all waste types during every phase of the waste management process in the operation of nuclear facilities.
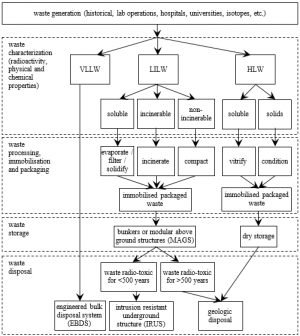
Properties of waste include:
- Radiological properties, e.g. half-life, activity and concentration of radionuclides, heat generation, decay products;
- Physical properties, e.g. solid, liquid and gaseous state, volume and weight, compactibility;
- Chemical properties, e.g. chemical composition and potential chemical hazard, solubility, combustibility and flammability, organic content, gas generation, corrosiveness;
- Biological properties such as bio-accumulation and potential biological hazards.
Examples of types of operational waste are dry solids, resins, sludges, slurry, aqueous or organic liquids, gases, metal, etc. The properties of wastes can be subdivided into (i) the properties of raw waste (as generated) that are essential to the determination of treatment methods, then (ii) the properties of treated waste that are essential for the selection of conditioning (stabilization) methods used for obtaining the waste form, and finally (iii) the properties of the waste form to select adequate packaging for disposal or storage. Process options include waste pretreatment, e.g. collection, segregation, and in situ decontamination, treatment of waste, e.g. volume reduction, removal of radionuclides from the waste, change of form by chemical process, etc., and waste conditioning, such as solidifying liquid waste in a glass, cement, bitumen or polymer, and putting the waste into a container suitable for transport and/or disposal.
Management of waste from generation to its end state, through various steps, should be considered in national radioactive waste management strategies[19]. Fig. 3 summarizes an example of a waste management strategy that has been prepared for Canada’s nuclear laboratories at Chalk River, Ontario and Whiteshell, Manitoba.
The discussion has so far touched upon wastes from mining and milling, reactor operation, and fuel reprocessing. Uranium bearing waste materials from uranium refining, conversion, enrichment and fuel manufacturing are extensively recycled either within the facility or to another facility for uranium recovery. Nonetheless, small volumes of radioactive waste arise that need to be placed into a safe end state.
As was noted in Section 2.1, the wastes arising from all components (facilities) of a NES need to be taken into account in performing a waste management assessment. Given the diversity of the wastes and related options for waste management, such an assessment will require input from a variety of technical specialists.
End states of nuclear waste
A given nuclear facility may generate a number of waste streams, and a management option will need to be defined for each stream to transfer the waste to its end state. End states include free or unconditional release, e.g. discharges of gas and liquids after they have been monitored to confirm that they meet discharge criteria agreed to with the responsible authority in the particular country. Other examples of free release may include release of decontaminated metals or other materials, again after monitoring to confirm that free release criteria are met. Waste management options also include recycling and /or reuse of materials, tools, equipment, etc., although in due course such material may appear again as a waste – for example when the plant is decommissioned. It is noted that monitored discharges and material reuse are often just part of ongoing operations and are often not thought of as options for waste management.
At present, there are a number of end states, i.e. disposal facilities, that have been licensed or are being developed. These include the following[20]:
“(a) Specific landfill disposal: Disposal in a facility similar to a conventional landfill facility for industrial refuse but which may incorporate measures to cover the waste. Such a facility may be designated as a disposal facility for VLLW with low concentrations or quantities of radioactive content[8]. Typical waste disposed of in a facility of this type may include soil and rubble arising from decommissioning activities. (b) Near surface disposal: Disposal in a facility consisting of engineered trenches or vaults constructed on the ground surface or up to a few tens of metres below ground level. Such a facility may be designated as a disposal facility for LLW[8]. (c) Disposal of intermediate level waste: Depending on its characteristics, ILW can be disposed of in different types of facility[8]. Disposal could be by emplacement in a facility constructed in caverns, vaults or silos at least a few tens of metres below ground level and up to a few hundred metres below ground level. It could include purposely built facilities and facilities developed in or from existing mines. It could also include facilities developed by drift mining into mountainsides or hillsides, in which case the overlying cover could be more than 100 m deep. (d) Geological disposal: Disposal in a facility constructed in tunnels, vaults or silos in a particular geological formation (e.g. in terms of its long term stability and its hydrogeological properties) at least a few hundred metres below ground level. Such a facility could be designed to receive HLW[8], including spent fuel if it is to be treated as waste. However, with appropriate design, a geological disposal facility could receive all types of radioactive waste. (e) Borehole disposal: Disposal in a facility consisting of an array of boreholes, or a single borehole, which may be between a few tens of metres up to a few hundreds of metres deep. Such a borehole disposal facility is designed for the disposal of only relatively small volumes of waste, in particular disused sealed radioactive sources. A design option for very deep boreholes, several kilometres deep, has been examined for the disposal of solid HLW and SNF, but this option has not been adopted for a disposal facility by any State. (f) Disposal of mining and mineral processing waste: Disposal usually on or near the ground surface, but the manner and the large volumes in which the waste arises, its physicochemical form and its content of long lived radionuclides of natural origin distinguish it from other radioactive waste. The waste is generally stabilized in situ and covered with various layers of rock and soil.”
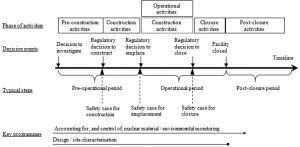
As stated in Ref[20], the term ‘disposal’ refers to the emplacement of radioactive waste into a facility or a location with no intention of retrieving the waste, although the possibility of retrieval is not ruled out (see Ref[6]).
The relatively large volume of mining and mineral processing waste, natural radionuclides such as 226Ra, and chemically toxic materials such as arsenic, impose constraints on the nature of the end states that can be practically utilized. Above ground mounds, water covers and pits[22][23][24][25] have been used in the past to isolate such wastes, but so-called pervious surround techniques[26][27][28] are more recently being applied. Although barriers can be incorporated into the designs of above grounds mounds to limit the release of contaminants, it is recognized that the proximity of wastes to the surface means that human intrusion remains a distinct possibility should institutional controls fail. Therefore, to limit the impact of such intrusion, care needs to be taken to reduce the concentrations of radioactivity in such facilities. Since pervious surround systems are constructed below the surface, they would seem to be less prone to inadvertent human intrusion, although such intrusion still remains a possibility.
It is noted that, as of 2019, disposal of VLLW, LLW and ILW was already an established industrial practice. In Ref[29], an overview of both proven and potential approaches for ILW disposal facilities is presented. Commercial operational experience does not yet exist for the disposal of HLW, although a license for construction of the spent nuclear fuel disposal facility in Finland was granted by the Finnish authority in 2015.
Geological disposal is defined[20] as the emplacement of solid radioactive waste in an underground facility in a stable geological formation (host rock). Three broad periods associated with the development – typically taking place over several decades – of a geological disposal facility can be identified: (i) the pre-operational period includes the definition of concepts, site investigation and selection, design studies and development of the safety case to receive regulatory approval of the construction; (ii) the operational period begins when waste is first received at the facility; and (iii) the post-closure period begins after all engineered containment and isolation features have been put in place, i.e. the facility has reached its final configuration. Fig. 4 illustrates these three development periods of a geological disposal facility.
The time line shown above is in principle also valid for all other types of waste disposal facilities discussed before[12][30].
Organisations for waste management
In many Member States, waste management activities are carried out by at least two different organizations/entities, namely the owner/operator of the facility in which the waste is generated, and the owner/operator of the end state facility. In some cases, on the waste generation side, or on the waste processing side, different operating divisions of the same entity could be involved, as could entirely different entities. The owner/operator of the facility in which the waste is generated will classify and segregate the wastes from that facility, and possibly process the waste to the disposal ready form and provide storage, before transferring the waste to the owner/operator of the end state facility for disposal.
In most cases, the owner/operator of the end state facility would take responsibility for siting, constructing, licensing, and operating the end state facility and then decommissioning it and placing it in its final configuration (called closure). That entity normally defines waste acceptance criteria based on the safety case for the end state facility and inventory of the existing and forecast waste. Waste acceptance criteria are usually discussed with waste generators/processors and approved by the responsible regulator. The owner/operator of the facility in which the waste arises will have to comply with waste acceptance criteria once these are accepted by all involved. In some cases, the operator of the end state facility may partially or completely process the wastes received.
Ref[31] provides detailed recommendations and guidance on the safety infrastructure – including that for radioactive waste management, spent fuel management and decommissioning – that needs to be established before deciding to launch a nuclear power programme and during the preparation for and construction of the first NPP.
Cost of waste management
In general, the costs of waste management are included in the price of the product that results in waste generation. Therefore, the cost of uranium from a given mine would be expected to include the costs that the mine owner incurs to manage the mine wastes. Similarly, the price that a fuel manufacturer charges, would be expected to include the cost that the manufacturer incurs in managing the associated wastes.
Where end state facilities exist, and wastes are being transferred to these facilities, the costs for waste management will reflect actual costs. In some situations, end state facilities may not yet be operating, most notably end state facilities for spent fuel (open fuel cycle) and HLW and long lived waste from the reprocessing of SNF (closed fuel cycle). In such situations, the operator of the facility in which the waste originates is expected to be working either with the entity responsible for establishing the end state facility or with the responsible body in the government that will represent the interests of the State and the public. The estimate, with appropriate contingencies and costs for placing the wastes in the end state, including the cost of establishing and operating the end state facility, should be included in the price of the product. A utility operating a power reactor on an open fuel cycle should include the cost of disposing of the spent fuel (as well as of its storage prior to disposal) in the price of electricity produced by the nuclear power plant.
Similarly, the operator of a reprocessing facility should include, one way or another, the cost of disposing of the associated wastes in the price charged. Today, reprocessing contracts normally require that the resulting HLW be returned to the customer, i.e. the utility that contracted for the reprocessing, so that the utility bears the cost of disposal for these wastes directly. On the other hand, the waste processing company normally retains the long-lived LILW and so includes the cost of disposing of this waste in the price charged to the utility for reprocessing. This means that the utility incurs the cost as an indirect cost. Regardless of the details, the price of electricity from a nuclear power plant will normally include a financial provision for the costs of managing its used fuel and/or reprocessing wastes, and will also include, indirectly, the costs of managing wastes from the front end of the fuel cycle as a fuel expense.
Necessary INPUT for a sustainability assessment in the area of waste management
This section defines the necessary input for an INPRO sustainability assessment in the area of waste management and the corresponding sources of information.
General description of necessary INPUT
The INPRO methodology user requirements for sustainability assessment in the area of waste management call for:
- Adequate classification of wastes to facilitate waste management (INPRO user requirement UR1);
- Waste to be minimized (UR1);
- Waste to be managed so that all intermediate steps in the waste management process are taken into account (UR2).
- Determination of end states for all classes of nuclear wastes and transfer of wastes to the end states as soon as practicable (UR3),
- Inclusion of the cost of all waste management activities in the estimated cost of energy from the nuclear energy system (UR3),
The basic task facing an INPRO assessor in the area of waste management is to determine, for each of the facilities that comprise the nuclear energy system (NES), whether:
- Wastes have been classified;
- There is evidence of waste minimization;
- End states have been defined for all wastes and there is evidence that wastes will be transferred to the specified end states as soon as practicable;
- Costs to manage waste are included in the cost of energy from the NES;
- All intermediate steps required to manage the waste have been taken into account.
As discussed in Section 2.2, management of a waste needs to be defined for a given waste that describes how the waste is to be managed from its generation to the placement of the waste in its end state. This means that the INPRO assessor needs descriptions of how wastes are managed (or proposed to be managed) for each of the facilities comprising the NES that is being assessed. These should contain the information needed by the assessor and they are supposed to be provided upon request to the assessor by the appropriate national organization if it is established (see Section 2.6) or by all parties involved in waste management activities (e.g. generator, processor and disposal operator).
An important feature of an effective and efficient waste management system is the existence of an adequate national system of classification and categorization for the processing of radioactive waste. The INPRO assessor needs to review that national system and confirm its adequacy.
In addition, the INPRO assessor needs to ascertain from the various organizations involved in the fuel cycle (e.g. mine operators, fuel manufacturers, and power plant operator) how the costs for management of waste are determined for each facility in the NES and whether they are reflected in the price of the associated products to ensure that the all of these costs have been accounted for.
Assessment INPUT needed for a country with experience of operating nuclear power plants
In conducting an INPRO sustainability assessment for a NES within a country that is already operating nuclear power plants, the assessor can generally expect to find that the country already has a well-developed set of waste management facilities and processes in place for each type of nuclear facility already operating in that country, such as mining facilities, fuel manufacturing facilities, nuclear power plants, etc, including end state facilities for short lived wastes. It can further be expected that the responsibilities of all parties involved are well understood and that waste management organizations are in place that have been given the responsibility for establishing end state facilities for all types of waste, including those for spent fuel declared as waste and/or HLW from reprocessing and for other long lived wastes.
The assessor would seek to obtain from each of the facilities in operation a description of the waste management practices being followed to determine whether they comply with the INPRO methodology requirements, or whether they would have to be modified. For example, if current operating reactors are using an open fuel cycle with plans for direct disposal of the spent fuel, and the NES being assessed is based on a closed fuel cycle which would necessitate reprocessing, the existing waste management plans would have to be modified to take into account the wastes from reprocessing. It would then be up to the designer of the NES to provide information on how the existing waste management practices would be modified to enable the assessor to complete the assessment.
It may also be the case that current waste management practices in some countries with operating nuclear power plants do not fully comply with the sustainability requirements in the INPRO methodology area of waste management. This would become clear as the assessor discusses and reviews waste management practices with the operators of the various facilities.
Assessment INPUT needed for a country planning its first nuclear power plant
For a country planning its first nuclear power plant, it is unlikely that waste management plans will already be in place. Therefore, as part of the planning for infrastructure[32], the issue of waste management will need to be addressed, and, in particular, responsibility will need to be assigned for siting, constructing and operating end state facilities for the waste from the nuclear power plant. This responsibility can be set out in the nuclear law or the government can use some other appropriate mechanism to establish a waste management policy. There are several possibilities. One is to assign responsibility to the owner/operator of the nuclear power plant, which may then create a dedicated division within its organization or establish a separate company to fulfil this responsibility. Another approach is for the government to retain responsibility for end state waste management facilities and to establish a dedicated government owned organization to discharge this responsibility but to recover the costs of doing so through a fee charged to the owner/operator of the nuclear power plant. In any case, an organization(s) responsible for end state facilities needs to be established as planning for a first nuclear power plant proceeds[31].
The owner/operator of the nuclear power plant will have to establish plans for managing the wastes produced by the power plant on an interim basis until the wastes are transferred to the operator of the end state facilities. The organization with responsibilities for the end state facilities will have to establish plans for siting, constructing and bringing these facilities into operation in due course. The operators of the nuclear power plant and of the end state facilities will need to coordinate their planning. End state facilities for operational radioactive waste from an NPP, other than HLW, need to be put into operation as soon as reasonably practicable, depending on the chosen waste management practice and availability of storage options at the plant site. The time taken to site, design, construct, and bring into operation a disposal facility for HLW (such as SNF declared as waste) is relatively long (usually a few decades) and the process is also relatively costly. Therefore, while considerations for such a HLW facility need to begin early in the process of planning for a first nuclear power plant, significant expenditures on implementing the plan would likely commence only after the first plant had entered into operation and provided a revenue stream to fund the implementation activities.
It is recommended that, for a first nuclear power plant, the INPRO assessment should initially focus on the waste from the power plant only. To simplify matters, it can further be assumed that an open fuel cycle will be used. Early on in the planning for a first nuclear power plant, it is likely that little evidence will be available for determining whether the INPRO methodology requirements in the area of waste management will be met. At such an early stage, the INPRO assessment in the area of waste management will serve primarily to identify gaps in knowledge and planning that will need to be addressed as the nuclear power programme proceeds. Subsequent INPRO assessments can be used to track progress. By the time the first nuclear power plant comes into operation, it would be expected that planning for managing the waste produced by the power plant, and implementation of these plans, would be sufficiently developed to support a conclusion that the INPRO methodology requirements will be met at least for the nuclear power plant. As experience is gained with the INPRO methodology, the scope of the INPRO assessment would be expanded to cover the other components of the NES, as required by the methodology, including, if necessary, reprocessing facilities and the management of long-lived wastes from reprocessing.
As stated before, it is assumed that an INPRO assessor in the area of waste management will have a general background in nuclear waste management. For a country that is just starting to plan for a first nuclear power plant, such an expert may not be available within the country. In that case, the country will need to assign responsibility for the INPRO assessment in the area of waste management to some organization. The selected organization could then very well become the lead organization for establishing the end state facilities and have a resulting need to develop its expertise.
This manual provides guidance on how to perform an INPRO assessment of NES sustainability in the area of waste management. Additionally, this publication contains general information on waste management strategies and planning that can be of particular assistance to countries that are new to radioactive waste management. However, the publication is not intended to serve as a general manual for waste management or as guidance on how to manage waste.
Summary of sources of information for an INPRO assessment
The task of an INPRO assessor in the area of waste management is largely to assemble information on waste management strategies, plans, and activities from the many different organizations that (will) operate the nuclear facilities, including the waste management facilities that comprise the NES being evaluated. Where such facilities exist, the information can be readily available from the facility operators. Where such facilities are under development, the developer of the NES is requested to provide the information needed by the assessor and provide assistance to the assessor in carrying out the assessment.
As noted in Section 2.1, an INPRO assessment in the area of waste management has to take into account waste generation and waste management practices in a wide range of facilities. It is doubtful that any single individual will be familiar with all of the facilities that need to be considered. It can be anticipated that information will need to be obtained from a variety of sources.
The IAEA has published many reports on waste management practices (e.g. Refs[6][19][29]) and safety standards (e.g. Refs[20][25][21][33]) and these publications may be consulted. IAEA experts in waste management in the Department of Nuclear Energy, and the Department of Nuclear Safety and Security may be consulted.
Much useful information can also be obtained from country reports submitted to the IAEA in accordance with the Joint Convention on the Safety of Spent Fuel Management and on the Safety of Radioactive Waste Management[34].
Additional information in the public domain can be obtained from proceedings of waste management conferences and from the web site publications of organizations operating nuclear fuel cycle facilities, including mining and milling, refining and conversion, fuel manufacturing, utilities operating nuclear power plants, reprocessing, and waste management organizations. These organizations are required to inform the general public on a regular basis about waste management activities carried out within the organizations as part of their operations, including, for example, steps taken to recycle and reuse materials to reduce the waste arisings.
An example of an assessment (using the INPRO methodology) of the waste management of a country embarking on a nuclear power programme is documented in Ref[35].
Valuable sources of information for an INPRO assessment may be found in the results of the various IAEA services offered to Member States in the area of Radioactive Waste Safety.
If a country is using the Integrated Nuclear Infrastructure Review (INIR) service from the IAEA in parallel with a nuclear energy system assessment (NESA) applying the INPRO methodology, the NESA team should contact the national Nuclear Energy Programme Installation Organization (NEPIO) involved in the INIR process to coordinate both activities and harmonize the results. For the INPRO methodology area of waste management, the results of INIR activities[36] regarding issue No. 16, radioactive waste could provide helpful input to the INPRO assessment, and vice versa.
INPRO basic principle, user requirements and criteria for sustainability assessment in the area of waste management
This section presents the INPRO methodology basic principle, user requirements and criteria for NES sustainability assessment in the area of waste management and notes how these relate to the Safety Fundamentals[37] and the Nuclear Energy Basic Principles[38].
Relationship of the safety fundamentals and nuclear energy principles to the INPRO methodology
The IAEA approach to strategic nuclear energy system planning involves assessing the sustainability of such systems based on the Brundtland definition of sustainable development. The INPRO methodology provides a tool for assessing the sustainability of a nuclear energy system. The methodology was developed to help identify potential gaps during the planning stages of the nuclear power programme. The manuals do not, however, address compliance with the IAEA Safety Standards or substitute a safety assessment to be performed as part of the licensing processes of NES facilities, but rather focus on the fulfilment of the INPRO methodology requirements in this area to achieve long term sustainability of the NES assessed. However, it is clear that the INPRO methodology manuals must assume licensing to be an absolutely necessary step in the development of any NES.
The INPRO methodology in the area of waste management was originally developed between 2001 and 2005 and was partly derived from the nine IAEA Fundamental Principles of Radioactive Waste Management[39] published in 1995. The Principles had formed the ethical and conceptual basis for the Joint Convention on the Safety of Spent Fuel Management and on the Safety of Radioactive Waste Management[34]. Many of the safety requirements and protection concepts adopted in the IAEA Safety Standards and in the Joint Convention[34] derive from the recommendations of the International Commission on Radiological Protection[40][41][42].
In 2006, the Principles of Radioactive Waste Management, the Safety of Nuclear Installations[43] (published in 1993) and Radiation Protection and the Safety of Radiation Sources[44] (published in 1996) were unified in a joint effort sponsored by Euratom, Food and Agriculture Organization of the United Nations, IAEA, International Labour Organization, International Maritime Organization, the Nuclear Energy Agency of the Organization for Economic Co-operation and Development, Pan American Health Organization, United Nations Environment Programme and World Health Organization, and published as the Fundamental Safety Principles[37].
INPRO requirements in the area of waste management are related to the Fundamental Safety Principle 7: Protection of present and future generations[37]. This Fundamental Safety Principle requires that people and the environment, present and future, must be protected against radiation risks. Part of the elaboration on this Fundamental Safety Principle provided in Ref[37] requires that “Radioactive waste must be managed in such a way as to avoid imposing an undue burden on future generations; that is, the generations that produce the waste have to seek and apply safe, practicable and environmentally acceptable solutions for its long term management. The generation of radioactive waste must be kept to the minimum practicable level by means of appropriate design measures and procedures, such as the recycling and reuse of material”.
Several INPRO user requirements and criteria for sustainability assessment in the area of waste management have apparent linkages to the following relevant safety requirements for predisposal waste management as published in Ref[45]:
“Requirement 8: Radioactive waste generation and control All radioactive waste shall be identified and controlled. Radioactive waste arisings shall be kept to the minimum practicable. … “Requirement 9: Characterization and classification of radioactive waste. At various steps in the predisposal management of radioactive waste, the radioactive waste shall be characterized and classified in accordance with requirements established or approved by the regulatory body. … “Requirement 10: Processing of radioactive waste. Radioactive material for which no further use is foreseen, and with characteristics that make it unsuitable for authorized discharge, authorized use or clearance from regulatory control, shall be processed as radioactive waste. The processing of radioactive waste shall be based on appropriate consideration of the characteristics of the waste and of the demands imposed by the different steps in its management (pretreatment, treatment, conditioning, transport, storage and disposal). Waste packages shall be designed and produced so that the radioactive material is appropriately contained both during normal operation and in accident conditions that could occur in the handling, storage, transport and disposal of waste. … “Requirement 12: Radioactive waste acceptance criteria. Waste packages and unpackaged waste that are accepted for processing, storage and/or disposal shall conform to criteria that are consistent with the safety case.”
In 2008, the IAEA published a document entitled Nuclear Energy Basic Principles[38]. The INPRO methodology area of waste management is linked to Principle No. 6 of that document, which states that “the use of nuclear energy should be based on a long term commitment”. Ref[46] sets out the objectives of radioactive waste management that support the Nuclear Energy Basic Principles.
Several issues related to safety and waste management are covered in separate volumes of the INPRO methodology. The issues of safety of nuclear fuel cycle facilities, including spent fuel reprocessing and storage facilities, are covered in the INPRO methodology manual on fuel cycle safety. Protection of humans and the environment from the stressors released and discharged from waste management facilities during normal operation and anticipated operational occurrences is covered in a separate area of the INPRO methodology dealing with environmental stressors.
INPRO Basic principle for sustainability assessment in the area of waste management
As stated in the Safety Fundamentals[37], a nuclear energy system (NES) requires measures that will protect human health and the environment from adverse effects of radioactive waste now and in the future. These measures should not impose undue burdens on future generations. These considerations are reflected in the INPRO methodology basic principle for sustainability assessment in the area of waste management, as set out below.
INPRO basic principle for sustainability assessment in the area of waste management: Radioactive waste in a NES is managed in such a way that it will not impose undue burdens on future generations.
This principle is based on the ethical consideration that the generations that receive the benefits of a practice are expected to bear the responsibility to manage the resulting waste. Limited actions, however, may be passed to succeeding generations, for example, the continuation of institutional control over a disposal facility, if and as needed.
The (planned) nuclear power programme should include provisions for the construction and operation of nuclear waste facilities, and provisions of funding to safely manage the waste in the future and safely dispose of it at an appropriate time. The (planned) management of the radioactive waste should, to the extent possible, not rely on long-term institutional arrangements as a necessary safety feature, recognizing that the reliability of such arrangements is expected to decrease with time.
INPRO has developed three user requirements, UR1 to UR3, for sustainability assessment in the area of waste management that elaborate on the INPRO basic principle formulated above. Two or more criteria were established for each of these INPRO methodology user requirements. The INPRO user requirements for sustainability assessment in the area of waste management are addressed primarily to the designer/developer together with the operator of the NES but also to the government that has responsibilities for nuclear waste management. The role of the INPRO assessor is to check via an assessment of the related criteria whether all parties involved have achieved what is asked for in the INPRO user requirements.
User requirement UR1: Classification, categorization and minimization of waste
INPRO user requirement UR1 for sustainability assessment in the area of waste management: The radioactive waste is classified and categorized to facilitate waste management in all parts of the NES, and the NES is designed and operated to minimize the generation of waste at all stages, with emphasis on waste containing long-lived radio-toxic components that would be mobile in a repository environment.
INPRO user requirement UR1 refers to optimization of the waste management process with respect to overall operational and long-term safety. The optimization will require a waste classification and categorization scheme that facilitates optimal management of various waste types within a NES. The scheme should be applicable to the entire fuel cycle.
Classification and categorization of radioactive waste provides a link between the waste characteristics and the requirements for waste management safety in the NES, particularly that of the end state. All wastes in the same category of the classification and categorization scheme are supposed to have a common end state (see the discussion of UR3 for a detailed description of the end state).
In keeping with the globally accepted principle of pollution prevention, the first INPRO user requirement, UR1, states that the generation of radioactive waste needs to be kept to a minimum practicable. Reduction of waste at the source is a preferred method that is consistent with the objectives of INPRO and one whose importance may grow if the global use of nuclear energy increases in the future.
The design stage offers the greatest potential for reducing waste as it offers the maximum flexibility to adjust the characteristics of the system for this purpose[47]. The minimization of waste by design is inherently safer than depending upon operational practices. It is particularly important to reduce components of the waste that are toxic for a long time and that are mobile in the repository (end state) environment. Mobile radioactive nuclides are the main contributors to the radiological impact of a repository on humans and the environment.
Methods for minimizing the radioactive waste include:
- Optimizing the design to reduce waste generation during operation and to facilitate decommissioning and dismantling of facilities[12][48];
- Segregation of waste streams to avoid cross contamination, to increase the proportion of waste suitable for controlled or free release, and to decrease the volume of material that represents a long-term hazard;
- Recycling and reuse of materials that would otherwise be radioactive waste;
- Extraction of long-lived decay products in mining and milling operations;
- Reduction of secondary waste from waste management processing and storage systems.
Technologies worthy of consideration for further development include (see also Appendix II):
- Improvement of both aqueous and non-aqueous methods of processing spent fuel;
- Partition and transmutation[49][50] of long-lived radionuclides in power reactors or accelerator driven systems;
- Application of advanced materials, such as cobalt-free steels, to reduce activation;
- Improved fuel cycle efficiency;
- Improved efficiency of the energy conversion process at reactors;
- Improved methods for processing different waste streams prior to packaging and disposal;
- Improved decontamination technology.
While the most desirable approach for reducing waste is to do so at the source, there are limitations on how much reduction at the source is possible while still operating effectively and economically. The waste that is produced can be treated to reduce the volume requiring conditioning to the end-form ready for disposal. Such reduction is already achieved in many facilities using current technologies, including:
- Compaction, super compaction, incineration, sintering and melting (for solids);
- Chemical precipitation, evaporation, ion exchange and membrane separation (for liquids);
- Solidification of liquid concentrates (cementation, bituminization, vitrification, drying).
New technologies for volume reduction are also being investigated such as:
- Cold crucible melting and plasma melting; and
- Non-flame technologies such as steam reforming, electron beam, UV photo-oxidation and supercritical waste oxidation.
For user requirement UR1, INPRO has defined the two criteria presented in Table 1.
Criterion CR1.1: Waste classification and categorization
ᅠIndicator IN1.1: Waste classification and categorization scheme.ᅠ
|
---|
Acceptance limit AL1.1: The scheme permits unambiguous, practical segregation for processing, storage and disposal, and identification of waste arisings.
Examples of Type 1 wastes include :
Examples of Type 2 wastes may include among others:
Some examples of Type 3 waste include:
For each of these types of waste, the waste can be further categorized based on its origin, process options, and radiological, physical, chemical and biological properties. |
Criterion CR1.2: Waste minimization
ᅠIndicator IN1.2: Characteristics of waste generated by the NES.ᅠ
|
||||
---|---|---|---|---|
Acceptance limit AL1.2: NES waste specific characteristics (mass, volume, total activity; amount of alpha-emitters, long-lived radionuclides and chemically toxic elements) have been minimized.
The associated acceptance limit AL1.2 (NES waste characteristics have been minimized) asks for a minimization study performed by the designer or by designer together with the operator as it is briefly outlined in Waste minimization study section. However, in situations when sufficient information is not available (e.g. in early stage of the design development or in early stage of nuclear power programme implementation) INPRO assessor can make a judgement using the outcome of a comparative study between the assessed NES and a standard NES as explained below.
Final assessment of CR1.2 waste minimization |
User requirement UR2: Predisposal waste management
INPRO user requirement UR2 for sustainability assessment in the area of waste management: Intermediate steps between generation of the waste and the end state are taken as early as reasonably practicable. The processes do not inhibit or complicate the achievement of the end state.
The basic steps in radioactive waste management: pretreatment, treatment, conditioning, storage, transportation, and disposal are expected to be considered in the planning for a NES.
The second INPRO user requirement, UR2, deals with the steps in predisposal waste management. By definition, the state of the waste that provides permanent safety without further modification is the end state. Other states of the waste that occur during operation of the fuel cycle are considered intermediate states leading to the end state. The waste has to be put in its end state by steps. Leaving these steps to future generations without compensating justification would fail to meet the INPRO basic principle for sustainability assessment in the area of waste management, which states that radioactive waste needs to be managed in such a way that will not impose undue burdens on future generations.
The implementation of different steps should not complicate the achievement of the end state. Care should be taken of the interdependences among all steps in predisposal waste management in order to avoid converting the waste into a form that is incompatible with planned subsequent steps[45]. Furthermore, waste should not be put into a form that would increase the difficulty of attaining the waste form planned for the end state. The safety of each process and activity (including transportation), under normal and accidental conditions, needs to be considered and all technical issues important for safety (e.g. removal of heat from the systems, storage in a sub-critical condition, properly confining the radioactive materials) need to be addressed.
Competing factors affect how soon the waste is brought to its final form for the end state. Early processing could preclude the use of potentially superior future technology. Delaying processing and final disposition could result in substantial near-term cost savings, but far greater weight has to be given to the decrease in uncertainty and increase in safety that will result from early achievement of an appropriate end state. The past practice, in some areas, of keeping high-level radioactive waste in liquid form, which is not appropriate in the long term, has led to a legacy of large amounts of such waste. This waste will now be subject to remediation at great cost to the present generation and could lead to significant accidental releases to the environment, as has happened on some occasions in the past. With an increase in the use of nuclear power it will become increasingly vital that waste be brought to a proper end state early. Retaining waste in forms and under conditions that are not permanently safe entails a risk that the waste will never be put into a proper state. The prescription ‘as early as reasonably practicable’ places significant weight on avoiding unnecessary delay.
Processing operations are part of the overall fuel cycle and their environmental and health effects need to be considered and justified by the net benefits that would be achieved by the processing step[58]. The ability to produce the waste form and package on an industrial scale should be evident, either through demonstration or confirmed conceptual design, before the nuclear energy system is implemented. This will give confidence that a fuel cycle would not generate waste for which the required end state is not feasible.
To demonstrate that the waste form is acceptable either the end state facility has been licensed or a safety case has been developed for a reference end state and the regulatory authority has indicated that the waste form considered for the reference end state is acceptable. In reality, such approval might only be provided, in principle, subject to the review of a detailed safety case prepared as part of a formal licensing application.
All technical issues for the safety of all processes and activities under normal and accidental conditions need to be taken into account and properly addressed. Such issues are strongly technology dependent and may change from one waste management strategy to another. For some processes, removal of decay heat may be required, in others, prevention of criticality may be an issue, or, in the transport of radioactive waste between two different processes, design of special casks might be required.
Factors important to sustainability in predisposal waste management include:
- Current and future quantities and potential hazards of the waste;
- Necessary degree of isolation of the waste;
- Dispersibility and mobility of the waste forms involved;
- Experience with, and maturity of, the technology, and potential for future advances;
- Reliability of equipment and its safety-related function;
- Complexity and degree of standardization of the activities;
- Novelty and maturity of the activity; and
- Organization size, number and complexity of interfaces and safety culture.
The form of the radioactive waste at the end of a process step has to be compatible with the next step, so effort needs to be made to ensure this in a large complex system. The design of the waste management system throughout the NES and throughout the life cycle of each of its components, needs to be seen as an integrated whole. Nothing should inhibit or complicate the achievement of the end state.
For user requirement UR2, INPRO has defined three criteria presented in Table 1.
Criterion CR2.1: Process descriptions
ᅠIndicator IN2.1: Process descriptions that encompass the entire waste life cycle.ᅠ
|
---|
Acceptance limit AL2.1: The complete chain of processes from generation to final end state is described in sufficient detail to make evident the feasibility of all steps. |
Criterion CR2.2: Time for waste form production
ᅠIndicator IN2.2: Time to produce the waste form specified for the end state.ᅠ
|
---|
Acceptance limit AL2.2: Consistent with the schedule for transfer of the waste to its end state. |
Criterion CR2.3: Predisposal waste management safety
ᅠIndicator IN2.3: Safety case for predisposal waste management facilities.ᅠ
|
---|
Acceptance limit AL2.3: Meets national regulatory standards and is consistent with applicable international safety standards. |
User requirement UR3 End state
INPRO user requirement UR3 for sustainability assessment in the area of waste management: An achievable end state that provides permanent safety without further modification is specified for each class of waste. The waste is brought to this end state as soon as reasonably practicable.
This requirement arises from the INPRO methodology basic principle for sustainability assessment in this area, which states that radioactive waste needs to be managed in such a way that it will not impose undue burdens on future generations. The end state is to protect people and the environment today from any harmful effects of the waste, and to protect people and the environment in the future to at least the same level that is acceptable today. The definition of an end state is supposed to include: the waste form and package; the final repository containing the waste packages; a safety case for the final repository; and a schedule for achieving the end state.
By definition, the state of the waste that provides permanent safety without further modification is the end state.
Ideally, the waste form and package are designed to contain radioactive materials until they have decayed to levels that meet the requirements for free release or for removal of regulatory control. In cases where this is not practicable, such as for long lived waste, other features of the waste management system have to be relied upon. The suitability of the waste form and package has to be proven in relation to the environmental conditions that they will be subjected to in the waste management scheme.
Low- and intermediate-level waste packages are isolated in relatively near surface repositories in many states. The protective features include the waste form and packages, sealing materials in the repository, as well as the natural barriers to movement of material through the geological environment.
Ultimately, the longer-lived components of waste will have to be put into a final waste form, packaged and the packages placed in some form of repository. The integrated system will have to be demonstrated to be permanently safe according to the current regulatory standards. The greatest emphasis today in national programs is to rely on underground repositories. The designs and operations of these facilities vary, e.g. in the depth at which packages are emplaced, the host geological medium chosen, and the period of monitoring prior to sealing and closure of the repository.
Most advanced nuclear power countries are planning to dispose of spent fuel and/or high-level waste from reprocessing of SNF in deeper repositories in stable geological media. Although progress is being made, it has proven difficult to site and license such a repository, so no repository for this waste is yet in operation and long-term storage is used in the interim.
In the case of a closed fuel cycle, the long-term safety of the final repositories could be improved by partitioning and transmutation involving the irradiation of long-lived radioisotopes to transform them into stable or short-lived elements[49][50]. This could significantly reduce the total amount of long-lived radioactive material requiring final disposal. Although the technology would require further development, it has the potential to significantly improve the long-term safety of radioactive waste from the fuel cycle.
In Ref[7], the term safety case is defined as “collection of arguments and evidence in support of the safety of a facility or activity”. It may relate to a given stage of development and, in such cases, it needs to acknowledge the existence of any unresolved issues and needs to provide guidance to resolve these issues in future development stages.
A minimum requirement is the determination that all applicable laws and regulations will be satisfied. The defined end state has to be permanently safe in the sense that future generations will not be exposed to risk that is not acceptable today. The safety case will need to include an analysis of any risks related to failure of institutional controls. It is expected that the safety case will be more easily developed for those end states that are primarily based on passive safety, i.e. where long-term institutional controls are not necessary for safety. If long-term institutional controls are necessary for safety, such as in the case of perpetual storage, the risk associated with potential failure of these controls needs to be accounted for in the safety case. It is recognized that, during the INPRO evaluation, a demonstration of safety would in general be impractical. The safety case would then need to rely on generally accepted theoretical analysis combined with evidence of component performance to the extent possible from present day operations of relevant facilities. For example, a waste form satisfying waste acceptance criteria of current disposal facilities would be acceptable.
The INPRO basic principle for sustainability assessment in the area of waste management states that radioactive waste is expected to be managed so as not to impose undue burdens on future generations. Therefore, people in the future need to be provided with the means to maintain the waste in a safe condition. The responsibility for providing these resources, including funds and proven technology, rests with those who have benefited from the generation of the waste, and the associated costs should be included in the estimated cost of energy. The internalization of all costs is a fundamental requirement of sound environmental management.
In principle, the assets accumulated to manage the waste are expected to cover the accumulated liability. This is contrary to the common practice of ‘under-funding’ the present liability and planning on the future value of money to compensate. Such a practice usually fails to properly internalize the cost associated with waste production. More importantly, the practice provides a built-in incentive to delay processing and safe disposal of the waste. Some common sense judgment will have to be used to target a reasonable period after start-up of the NES in which to balance the assets and liabilities, because, otherwise, the liability associated with the first small generation of waste would be cost prohibitive. It should be understood that the cost of any long-term institutional controls associated with waste management needs to be included in the estimated cost of the NES.
For user requirement UR3 INPRO has established four criteria presented in Table 1.
Criterion CR3.1: End state technology
ᅠIndicator IN3.1: Availability of end state technology.ᅠ
|
---|
Acceptance limit AL3.1: End states are identified for all waste streams and all required technology is currently available or reasonably expected to be available on a schedule compatible with the schedule for introducing the waste management for all NES facilities. |
Criterion CR3.2: Safety of end state
ᅠIndicator IN3.2: Safety case for the end state.ᅠ
|
---|
Acceptance limit AL3.2: Meets regulatory standards and is consistent with applicable international safety standards. |
Criterion CR3.3: Schedule for achieving end state
ᅠIndicator IN3.3: Time to reach the end state.ᅠ
|
---|
Acceptance limit AL3.3: As short as reasonably practicable.
Otherwise, the judgment is that the acceptance limit AL3.3 has not been met. |
Criterion CR3.4: Resources for achieving end state
ᅠIndicator IN3.4: Availability of resources.ᅠ
|
---|
Acceptance limit AL3.4: Resources (funding, space, capacity, etc) are available for achieving the end state, compatible with the size and growth rate of the NES. Costs of all waste management steps are included as a specific line item in the product’s cost estimate. |
Appendix I
ᅠConcept of ALARPᅠ
|
---|
The concept of ALARP is mentioned in the INPRO methodology areas of waste management and environmental impact of stressors. The concept is illustrated in Fig. 6. The risk (symbolized by the triangle) is divided into three regions: a broadly acceptable region, a tolerable region where a process for ALARP has to be used, and an unacceptable region. ![]() FIG. 6. The concept of ALARP[70] As a first step of the ALARP concept to be applied within the INPRO methodology, the boundary values of these three regions have to be defined, such as the boundary between the tolerable and the unacceptable region, sometimes called a basic limit, and the boundary between the tolerable and broadly accepted region, sometimes called a basic objective. The next step is to confirm that the value of the indicator of a NES is within the ALARP region, which is below the basic limit and above the basic objective. The third step is to perform an optimization analysis to confirm that all measures to reduce the specific risk have been taken into account up to a level where the costs for these measures become grossly disproportionate to the benefit gained. It is important to note that, in case the indicator of a NES has a value in the broadly accepted region below the boundary basic objective, no further work is necessary to be performed to fulfil the ALARP concept. |
Appendix II
Example of radioactive waste inventories of a NES
This Appendix is based on information documented in an IAEA publication[71] that evaluated the global inventories of radioactive waste and other materials produced in nuclear energy systems (NES) up to 2007, and on results of the MIT study The Future of Nuclear Power[72]. It presents the normalized (to 1 GW∙a of electricity generated) mass flows in a NES from mining to disposal of radioactive material.
Front end of fuel cycle
The following Fig. 7 shows the fuel production chain (or front end of the nuclear fuel cycle) of a light water reactor (LWR) using enriched uranium fuel, including its waste arisings starting from uranium mining (in-situ leaching and strip or underground mining) and continuing through the delivery of fresh enriched uranium fuel assemblies to the nuclear power plant (NPP).
As shown in Fig. 7, all nuclear facilities of the LWR fuel chain produce nuclear waste with different mass, volume and radioactivity. The biggest volume and mass of waste with relatively low activity is produced in the uranium mine and milling facility. The conversion and fuel production facility generate small volumes and masses of waste with low activity. The enrichment plant produces a small amount of waste but a large mass of depleted uranium, which is strictly speaking not a waste as it is partly used in industrial applications and can be used in an advanced NES with mixed oxide (MOX) fuel. The nuclear power plant generates several types of operational waste and spent nuclear fuel (SNF) of relatively low mass and volume but with very high radioactivity. Typically, the SNF is kept in storage (on or off site the NPP) for several decades. Thereafter, in an open (or once through) fuel cycle the SNF is declared as HLW, conditioned and disposed of in a repository (the end state). In a closed fuel cycle, the SNF is transported to a reprocessing facility where fissile/ fertile material such as uranium and plutonium is recycled. The separated fissile/ fertile material is then used to produce new MOX fuel. The rest of the reprocessed SNF, mainly fission products and minor actinides (and secondary waste from reprocessing), is currently conditioned and disposed of as HLW. An estimation of the material balance of the nuclear fuel chain for electricity production of 1 GW∙a in a LWR is shown in Table 2. The numerical values of production and waste were calculated by using the on-line WISE calculator [73].
Facility | Product | Waste | ||
---|---|---|---|---|
Form | Value | Form | Amount | |
U mine | U ore (t) | 108 219 | Waste rock (t) | 541 097 |
U mill | U3O8 (t) | 244.4 | Tailings solids (t) | 107 975 |
Tailings liquid (m3) | 107 975 | |||
Conversion | UF6 (natural) (t) | 305.0 | Solid waste (t) | 144.4 |
Liquid waste (m3) | 1340.5 | |||
Enrichment | UF6 (enriched) (t) | 38.0 | Depleted U (t) | 267.0 |
Fuel fabrication | UO2 (assemblies) (t) | 28.86 | Solid waste (m3) | 12.7 |
Liquid waste (m3) | 228.9 | |||
NPP | Electricity (GW∙a) | 1 | Spent fuel (t) | 28.86 |
The estimated values of the material balance presented in Table 2 depend strongly on the characteristics of the fuel cycle facilities and the reactor selected for the calculation of materials produced and waste masses. Input parameters used for the calculation of results in Table 2 are shown in the following Table 3 for completeness.
Facility | Parameters | Values |
---|---|---|
U mine | Waste/ore ratio | 5 |
Ore grade (%) | 0.2 | |
U mill | Extraction losses (%) | 4.24 |
Solids in tailings effluent (%) | 50 | |
Conversion | Losses (%) | 0.5 |
Solid waste (t/tU) | 0.7 | |
Liquid waste (m3/tU) | 6.5 | |
Enrichment | Product assay (%) | 3.6 |
Tails assay (%) | 0.3 | |
Fuel fabrication | Losses (%) | 1 |
Solid waste (m3/tU) | 0.5 | |
Liquid waste (m3/tU) | 9 | |
NPP | Fuel burnup (GWd/tU) | 42 |
Efficiency (%) | 34.2 |
Changing from enriched uranium (3.6 % 235U) to natural uranium (0.71 % 235U) as fuel (by eliminating the enrichment step) and reducing the fuel burnup from 42 to 8 GWd/tU, the demand for natural uranium (needed for 1 GW∙a electricity production) is reduced from 206 to 134 t Unat, i.e. by almost 35 %, together with the waste arisings of mining, milling, and conversion. In this case, the demand for fresh fuel and the generation of SNF is quintupled (from 29 to 151 t SNF). However, the total activity of the SNF per GW∙a is expected to be about the same despite the difference in mass and volume, since the activity is, to a first approximation, proportional to the electricity produced. Use of natural uranium as fuel with a burnup of less than 10 GWd/tU is typical for heavy water moderated reactors. The radioactivity level of waste is dependent on the type of nuclear facility. The largest mass and volume of waste is generated in the mining and milling step of the nuclear fuel cycle. The tailings produced in milling have a specific activity (230Th and 226Ra) of 0.033 GBq/m3 assuming an average tailing density of 1.5 t/m3. The radioactivity level in mine residues is assumed to be lower by a factor of 10 compared to mill tailings. The total activity of mine residues (541097 t) and milling tailings (107975 t) for the production of 1 GW∙a electricity in a LWR (Table 2) is about 1.2 TBq and 2.4 TBq, respectively. The fuel cycle steps conversion, enrichment and fuel production generate radioactive waste in rather small quantities (see Table 2) compared to mining and milling facilities and with low (but long lived) radioactivity levels compared to the operation of an NPP, and (in the case of a closed fuel cycle) to a reprocessing facility and are therefore not considered further in this appendix.
Waste produced during Operation of an NPP
The operation of an NPP produces several classes of radioactive waste (see Fig. 2 in Section 2.4 of this report). For example, the typical (historical) annual operational LILW of a pressurized water reactor (PWR) and a boiling water reactors (BWR) generating 1 GW∙a of electricity is about 250 m3 with 100 TBq and 500 m3 with 500 TBq, respectively[71]. An HWR produces about the same amount of LILW as a PWR and WWER reactors produce a similar amount of LILW as BWRs.
The SNF from a nuclear power plant has a relatively small volume , but contains a high amount of radioactivity. To generate 1 GW∙a of electricity an LWR – as shown above in Table 2 – about 29 tHM of SNF (with a burn-up of 42 GWd/tU) are unloaded from the reactor core to be stored on site of the plant. LWR SNF having 1 tHM has a volume of about 0.74 m3 and an initial activity level of 1.6·105 TBq. For the production of 1 GW∙a electricity the SNF (with a mass of 29 tHM) has a volume of 21.5 m3 and contains about 5·106 TBq of total initial activity. During storage the activity of the SNF decreases due to decay of radioactive nuclides.
Due to the use of natural uranium as fuel the mass and volume of SNF fuel for 1 GW∙a produced is higher in a HWR compared to a LWR. As presented above, the mass of SNF is about 151 t. Assuming a mass of about 20 kg and a volume of about 0.005 m3 per HWR fuel bundle results in a total volume of about 40 m3. The total amount of radioactivity in HWR SNF is approximately the same as in LWR SNF for 1 GW∙a produced.
Back end of the fuel cycle
There are several options for the back end of a nuclear fuel cycle, i.e. an open fuel cycle, and a closed fuel cycle with different levels of closure. In a NES with an open (or once through) fuel cycle, the spent fuel will be disposed of as HLW. Fig. 8 shows a schematic of an open fuel cycle.
The annual demand for natural uranium for 1 GW∙a of produced electricity is estimated in Table 2 as 207 tU (or 244.4 t of U3O8). The total amount of SNF is ca. 29 tHM per 1 GW∙a. Applying the same conversion factor of 0.74 m3 per ton of HM in SNF as (see previous section), the annual volume of SNF per 1 GW∙a would be about 21.5 m3. Before the SNF is put in its end state (into a deep geological repository) the fuel elements have to be encapsulated in special containers. The encapsulation of SNF into disposal canisters increases the waste volume from 21.5 m3 to about 75 m3 of HLW.
The volume of encapsulated SNF from an HWR producing 1 GW∙a would increase from 40 to about 94 m3, assuming a container with a volume of about 4.5 m3 (4 m high, diameter of 1,2 m) storing 360 HWR spent fuel bundles.
As indicated above, the fuel cycle can be (partly) closed by (mono and multi) recycling of plutonium (and uranium) in SNF. Fig. 9 shows the example of a NES with a thermal reactor with mono recycling of all uranium fuel to separate plutonium for use in thermal reactor cores fuelled with about 30 % MOX and 70 % uranium oxide (UOX). Spent MOX fuel is disposed of as waste.
Compared to the open fuel cycle (Fig. 8), the use of mono recycling (Fig. 9) reduces the demand of natural uranium by about 15 % . In addition, if the separated uranium (ca. 94% of original uranium from fresh UOX fuel) is not defined as waste but as a resource then the remaining material to be disposed of as HLW consists only of spent MOX fuel elements and vitrified HLW (from reprocessing the uranium fuel elements). This may represent a significant reduction of HLW to be disposed of in comparison to an open fuel cycle[72].
Fig. 10 shows an example of a NES consisting of fast and thermal reactors and recycling of both UOX and MOX fuel. Theoretically, depending on the capacity of reactors involved and on the characteristics of the fast reactor, the demand for natural uranium and the amount of HLW can be reduced in such a NES over a broad range compared to a once through fuel cycle.
In the case of a (partly) closed nuclear fuel cycle, a reprocessing facility is needed to separate the fissile material in the SNF to produce MOX fuel. Currently, industrial reprocessing facilities apply the PUREX process to separate plutonium and uranium from the SNF. International research is performed to develop the separation process further enabling additional recycling of minor actinides and specific fission products.
Reprocessing of SNF of 1 tHM generates about 0.12 m3 of vitrified HLW[74]. If the complete uranium SNF of 29 tHM (used to generate 1 GW∙a in a LWR reactor, see Table 2) is reprocessed, about 3.5 m3 of vitrified HLW (glass) is produced, which is equivalent to about 25 m3 disposal volume after placement into disposal canisters . The total activity of the vitrified waste is estimated to be 3.7·104 TBq[71].
As mentioned above, the PUREX process is being used as a reprocessing technique and research is going on into other methods for effective separation of actinides. Research is also under progress in various countries on the development of a non-aqueous reprocessing method, based on molten salt electrorefining, called PYRO processing. In this process minor actinides remain with Pu and therefore no special minor actinides recycling method is required. Minor actinides will form part of the fast reactor fuel and eventually will get burned, e.g. in the fast reactors fuelled with metallic fuel which will be able to reduce the total amount of minor actinides produced in U-Pu closed fuel cycle. Another advantage of this process from waste management point of view is that no significant amounts of liquid HLW are expected to be generated during reprocessing. Besides that, the breeding capability of metal fuel FR can provide faster growth of NESs.
Decommissioning waste of nuclear facilities
It is reported in Ref[71] that the decommissioning of an LWR with a capacity of 1 GWe will generate a quantity of short lived LILW of about 6000 metric tons and less than 1000 metric tons of HLW and long lived LILW. Decommissioning of reprocessing plants is expected to produce an amount of radioactive waste similar to a nuclear power station but with a significantly higher fraction of long lived waste.
Summary of waste arising in a NES
Based on the information provided in Sections of this Appendix, the waste arisings (average values of historic data) of a standard NES with different types of reactors generating 1 GW∙a of electricity can be summarized in Table 4.
Waste Type | Mass (t) | Volume (m3) | Activity (TBq) | |||
---|---|---|---|---|---|---|
LWR | HWR | LWR | HWR | LWR | HWR | |
Mine residues (waste rock) | 5.4∙105 | 3.5∙105 | 3∙105 | 1.9∙105 | 1.2 | 0.77 |
Mill tailings | 1.1∙105 | 0.7∙105 | 1.1∙105 | 0.7∙105 | 2.4 | 1.5 |
LILW from reactor operation | 2.5∙102 | 2.0∙102 | 1.0∙102 | 1.0∙102 | ||
SNF (if disposed of) | 29 | 151 | 21.5 (FA) 75 (packed) |
40 (FA) 94 (packed) |
5∙106 | 5∙106 |
Vitrified HLW (if SNF reprocessed) | 3.5 (glass) 25 (packed) |
3.7∙104 | ||||
Short-lived LILW from decommissioning | 6000 | |||||
Long-lived LILW and HLW from decommissioning | <1000 |
[ + ] Assessment Methodology | |||||
---|---|---|---|---|---|
|
References
- ↑ 1.0 1.1 1.2 INTERNATIONAL ATOMIC ENERGY AGENCY, INPRO Methodology for Sustainability Assessment of Nuclear Energy Systems: Environmental Impact of Stressors, IAEA Nuclear Energy Series No. NG-T-3.15, IAEA, Vienna (2016).
- ↑ 2.0 2.1 2.2 2.3 2.4 INTERNATIONAL ATOMIC ENERGY AGENCY, Guidance for the Application of an Assessment Methodology for Innovative Nuclear Energy Systems, INPRO Manual, IAEA-TECDOC-1575/Ref.1, Vienna (2008).
- ↑ 3.0 3.1 3.2 3.3 UNITED NATIONS, Our Common Future (Report to the General Assembly), World Commission on Environment and Development, UN, New York (1987).
- ↑ INTERNATIONAL ATOMIC ENERGY AGENCY, Viability of Sharing Facilities for the Disposal of Spent Fuel and Nuclear Waste, IAEA-TECDOC-1658, IAEA, Vienna (2011).
- ↑ INTERNATIONAL ATOMIC ENERGY AGENCY, Framework and Challenges for Initiating Multinational Cooperation for the Development of a Radioactive Waste Repository, IAEA Nuclear Energy Series No. NW-T-1.5, IAEA, Vienna (2016).
- ↑ 6.0 6.1 6.2 INTERNATIONAL ATOMIC ENERGY AGENCY, Geological Disposal of Radioactive Waste; Technological Implications for Retrievability, IAEA Nuclear Energy Series No. NW-T-1.19, IAEA, Vienna (2009).
- ↑ 7.0 7.1 INTERNATIONAL ATOMIC ENERGY AGENCY, IAEA Safety Glossary, Terminology used in Nuclear Safety and Radiation Protection, 2018 Edition, IAEA, Vienna (2019).
- ↑ 8.0 8.1 8.2 8.3 8.4 8.5 8.6 8.7 INTERNATIONAL ATOMIC ENERGY AGENCY, Classification of Radioactive Waste, IAEA Safety Standards Series No. GSG-1, IAEA, Vienna (2009)
- ↑ 9.0 9.1 INTERNATIONAL ATOMIC ENERGY AGENCY, Application of the Concepts of Exclusion, Exemption and Clearance, IAEA Safety Standards Series No. RS-G-1.7, IAEA, Vienna (2004).
- ↑ INTERNATIONAL ATOMIC ENERGY AGENCY, Disposal Aspects of Low and Intermediate Level Decommissioning Waste, Results of a coordinated research project 2002 – 2006, IAEA-TECDOC-1572, IAEA, Vienna (2007).
- ↑ INTERNATIONAL ATOMIC ENERGY AGENCY, Managing Low Radioactivity Material from the Decommissioning of Nuclear Facilities, IAEA Technical Reports Series No. 462, IAEA, Vienna (2008).
- ↑ 12.0 12.1 12.2 INTERNATIONAL ATOMIC ENERGY AGENCY, Policies and Strategies for the Decommissioning of Nuclear and Radiological Facilities, IAEA Nuclear Energy Series No. NW-G-2.1, IAEA, Vienna (2011).
- ↑ USNRC, Licensing Requirement for Land Disposal of Radioactive Waste, Code of Federal Regulations, Title 10, Part 61, Washington (1992).
- ↑ FRENCH MINISTRY FOR INDUSTRY AND RESEARCH, Surface Centres for Long Term Disposal of Radioactive Waste with Short or Medium Half-Life and with Low or Medium Specific Activity, Basic Safety Regulations, Regulation No. 12, Paris (1984).
- ↑ KIM, J.I., et al, German Approaches to Closing the Nuclear Fuel Cycle and Final Disposal of HLW, Corrosion Behavior of Spent Fuel, Proc. Int. Workshop Überlingen, 1995, Journal of Nuclear Materials No. 238 (1996) 1-10.
- ↑ INTERNATIONAL ATOMIC ENERGY AGENCY, Spent Fuel Reprocessing Options, IAEA-TECDOC-1587, IAEA, Vienna (2008).
- ↑ INTERNATIONAL ATOMIC ENERGY AGENCY, Categorizing Operational Radioactive Wastes, IAEA-TECDOC-1538, IAEA, Vienna (2007).
- ↑ INTERNATIONAL ATOMIC ENERGY AGENCY, Strategy and Methodology for Radioactive Waste Characterization, IAEA-TECDOC-1537, IAEA, Vienna (2007).
- ↑ 19.0 19.1 INTERNATIONAL ATOMIC ENERGY AGENCY, Policies and Strategies for Radioactive Waste Management, IAEA Nuclear Energy Series No. NW-G-1.1, IAEA, Vienna (2009).
- ↑ 20.0 20.1 20.2 20.3 20.4 INTERNATIONAL ATOMIC ENERGY AGENCY, Disposal of Radioactive Waste, IAEA Safety Standards Series No. SSR-5, IAEA, Vienna (2011).
- ↑ 21.0 21.1 21.2 INTERNATIONAL ATOMIC ENERGY AGENCY, Geological Disposal Facilities for Radioactive Waste, IAEA Safety Standards Series No. SSG-14, IAEA, Vienna (2011).
- ↑ ACOTT, P., and POLLOCK, R., Cluff Lake Decommissioning Project - Planning and Current Status, Proc. Canadian Nuclear Society Conference - Waste Management, Decommissioning and Environmental Restoration for Canada’s Nuclear Activities: Current Practices and Future Needs, Ottawa, ON, May 8-11, CNS (2005).
- ↑ HAGEN, H. and JAKUBICK, A.T., Environmental Remediation of the Wismut Legacy and Utilization of the Reclaimed Areas, Waste Rock Piles and Tailings Ponds, Proc. Canadian Nuclear Society Conference - Waste Management, Decommissioning and Environmental Restoration for Canada’s Nuclear Activities: Current Practices and Future Needs, Ottawa, ON, May 8-11, CNS (2005).
- ↑ LUDGATE, I., PRIMEAU, P., LAIBERTE, R, PEDLAR, R., The Decommissioning of the Denison Uranium Facilities in Elliot Lake, Proc. Canadian Nuclear Society Conference - Waste Management, Decommissioning and Environmental Restoration for Canada’s Nuclear Activities: Current Practices and Future Needs, Ottawa, ON, May 8-11, CNS (2005).
- ↑ 25.0 25.1 25.2 INTERNATIONAL ATOMIC ENERGY AGENCY, Management of Radioactive Waste from the Mining and Milling of Ores, IAEA Safety Standards Series No. WS-G-1.2, IAEA, Vienna (2002).
- ↑ FROST, S.E., Tailing Management for the 21st century, Proceedings of the 36th Annual Canadian Nuclear Association Conference, Fredericton, N.B., June 9-12, (1996).
- ↑ DE BOURAYNE, A., POLLOCK, R. AND J. ROWSON, J., Planning Ahead: Tailings Management for High-Grade Uranium Ores with High Arsenic and Nickel Content, Journal of Radwaste Solutions, May/June, p 42, (2000).
- ↑ TREMBLAY, M. AND ROWSON, J., Tailings Management Best Practice: A Case Study of the McClean Lake Jeb Tailings Management Facility, Proc. Canadian Nuclear Society Conference - Waste Management, Decommissioning and Environmental Restoration for Canada’s Nuclear Activities: Current Practices and Future Needs, Ottawa, ON, May 8-11, CNS (2005).
- ↑ 29.0 29.1 INTERNATIONAL ATOMIC ENERGY AGENCY, Disposal Approaches for Long Lived Low and Intermediate Level Radioactive Waste, IAEA Nuclear Energy Series No. NW-T-1.20, IAEA, Vienna (2009).
- ↑ INTERNATIONAL ATOMIC ENERGY AGENCY, The Management System for the Development of Disposal Facilities for Radioactive Waste, IAEA Nuclear Energy Series No. NW-T-1.2, Vienna (2011).
- ↑ 31.0 31.1 INTERNATIONAL ATOMIC ENERGY AGENCY, Establishing the Safety Infrastructure for a Nuclear Power Programme, IAEA Safety Standards Series No. SSG-16, IAEA, Vienna (2012).
- ↑ INTERNATIONAL ATOMIC ENERGY AGENCY, Milestones in the Development of a National Infrastructure for Nuclear Power, IAEA Nuclear Energy Series No. NG-G-3.1 (Rev.1), Vienna (2015).
- ↑ INTERNATIONAL ATOMIC ENERGY AGENCY, Near Surface Disposal Facilities for Radioactive Waste, IAEA Safety Standards Series No. SSG-29, IAEA, Vienna (2014).
- ↑ 34.0 34.1 34.2 34.3 INTERNATIONAL ATOMIC ENERGY AGENCY, Joint Convention on the Safety of Spent Fuel Management and on the Safety of Radioactive Waste Management, IAEA Information Circular INFCIRC/546, IAEA, Vienna (1997).
- ↑ INTERNATIONAL ATOMIC ENERGY AGENCY, INPRO Assessment of the Planned Nuclear Energy System of the Republic of Belarus, A report of the International Project on Innovative nuclear reactors and fuel cycles (INPRO), IAEA-TECDOC-1716, Vienna (2013).
- ↑ INTERNATIONAL ATOMIC ENERGY AGENCY, Evaluation of the Status of National Nuclear Infrastructure Development, IAEA Nuclear Energy Series No. NG-T-3.2 (Rev.1), Vienna (2016).
- ↑ 37.0 37.1 37.2 37.3 37.4 EUROPEAN ATOMIC ENERGY COMMUNITY, FOOD AND AGRICULTURE ORGANIZATION OF THE UNITED NATIONS, INTERNATIONAL ATOMIC ENERGY AGENCY, INTERNATIONAL LABOUR ORGANIZATION, INTERNATIONAL MARITIME ORGANIZATION, OECD NUCLEAR ENERGY AGENCY, PAN AMERICAN HEALTH ORGANIZATION, UNITED NATIONS ENVIRONMENT PROGRAMME, WORLD HEALTH ORGANIZATION, Fundamental Safety Principles, IAEA Safety Standards Series No. SF-1, IAEA, Vienna (2006).
- ↑ 38.0 38.1 INTERNATIONAL ATOMIC ENERGY AGENCY, Nuclear Energy Basic Principles, IAEA Nuclear Energy Series No. NE-BP, Vienna (2008).
- ↑ INTERNATIONAL ATOMIC ENERGY AGENCY, Principles of Radioactive Waste Management, Safety Fundamentals, IAEA Safety Series No. 111-F, IAEA, Vienna (1995).
- ↑ INTERNATIONAL COMMISSION ON RADIOLOGICAL PROTECTION, Radiological Protection Recommendations as Applied to the Disposal of Long-lived Solid Radioactive Waste, Publication 81, Elsevier Science, Oxford (1998).
- ↑ INTERNATIONAL COMMISSION ON RADIOLOGICAL PROTECTION, Radiological Protection Policy for the Disposal of Radioactive Waste, Publication 77, Elsevier Science, Oxford (1998).
- ↑ INTERNATIONAL COMMISSION ON RADIOLOGICAL PROTECTION, The 2007 Recommendations of the International Commission on Radiological Protection, Publication 103, Elsevier Science, Oxford (2007).
- ↑ INTERNATIONAL ATOMIC ENERGY AGENCY, The Safety of Nuclear Installations, IAEA Safety Series No. 110, IAEA, Vienna (1993).
- ↑ FOOD AND AGRICULTURE ORGANIZATION OF THE UNITED NATIONS; INTERNATIONAL ATOMIC ENERGY AGENCY; INTERNATIONAL LABOUR ORGANIZATION, OECD NUCLEAR ENERGY AGENCY, PAN AMERICAN HEALTH ORGANIZATION, WORLD HEALTH ORGANIZATION, Radiation Protection and the Safety of Radiation Sources, IAEA Safety Series No. 120, IAEA, Vienna (1996).
- ↑ 45.0 45.1 INTERNATIONAL ATOMIC ENERGY AGENCY, Predisposal Management of Radioactive Waste, IAEA Safety Standards Series No. GSR Part 5, IAEA, Vienna (2009).
- ↑ INTERNATIONAL ATOMIC ENERGY AGENCY, Radioactive Waste Management Objectives, IAEA Nuclear Energy Series No. NW-0, IAEA, Vienna (2011).
- ↑ 47.0 47.1 INTERNATIONAL ATOMIC ENERGY AGENCY, Consideration for Waste Minimization at the Design Stage of Nuclear Facilities, Technical Reports Series No. 460, IAEA, Vienna (2007).
- ↑ 48.0 48.1 INTERNATIONAL ATOMIC ENERGY AGENCY, Methods for the Minimization of Radioactive Waste from Decontamination and Decommissioning of Nuclear Facilities, Technical Reports Series No. 401, IAEA, Vienna (2001).
- ↑ 49.0 49.1 INTERNATIONAL ATOMIC ENERGY AGENCY, Implications of Partitioning and Transmutation in Radioactive Waste Management, Technical Reports Series No. 435, IAEA, Vienna (2004).
- ↑ 50.0 50.1 INTERNATIONAL ATOMIC ENERGY AGENCY, Assessment of Partitioning Processes for Transmutation of Actinides, IAEA-TECDOC-CD-1648, IAEA, Vienna (2010).
- ↑ INTERNATIONAL ATOMIC ENERGY AGENCY, Regulatory Control of Radioactive Discharges to the Environment, IAEA Safety Standards Series No. GSG-9, IAEA, Vienna (2018).
- ↑ 52.0 52.1 52.2 INTERNATIONAL ATOMIC ENERGY AGENCY, Recycle and reuse of materials and components from waste streams of nuclear fuel cycle facilities, IAEA-TECDOC-1130, IAEA, Vienna (2000).
- ↑ INTERNATIONAL ATOMIC ENERGY AGENCY, Minimization of waste from uranium purification, enrichment and fuel fabrication, IAEA-TECDOC-1115, IAEA, Vienna (1999).
- ↑ GOVERNMENT OF CANADA, Canadian National Report for the Joint Convention on the Safety of Spent Fuel Management and on the Safety of Radioactive Waste Management. Final Report (2011).
- ↑ NUCLEAR DECOMMISSIONING AUTHORITY, Monitoring Our Environment, Discharges and Environmental Monitoring Annual Report 2013. Sellafield Ltd, Seascale, United Kingdom (2014)
- ↑ ENVIRONMENT AGENCY, The Environmental Permitting (England and Wales) Regulations 2010. Environment Agency, Bristol (2010).
- ↑ ALLAN, C.J., BAUMGARTNER, P., Back-end of the Nuclear Fuel Cycle: A Comparison of the Direct Disposal and Reprocessing Options, Proceedings of the 10th Pacific Basin Nuclear Conference, Kobe, Japan, 20-25 October, 1996, Vol. 1, Page 51 (1996).
- ↑ INTERNATIONAL ATOMIC ENERGY AGENCY, New Developments and Improvements in Processing of ‘Problematic’ Radioactive Waste, Results of a coordinated research project 2003 – 2007, IAEA-TECDOC-1579, IAEA, Vienna (2007).
- ↑ 59.0 59.1 59.2 NAGRA, Project Opalinus Clay Safety Report Demonstration of Disposal Feasibility for Spent Fuel, Vitrified High-level Waste and Long-lived Intermediate Level Waste (Entsorgungsnachweis), Nagra Technical Report NTB 02-05, Nagra, Wettingen, Switzerland, (2002).
- ↑ 60.0 60.1 INTERNATIONAL ATOMIC ENERGY AGENCY, INPRO Methodology for Sustainability Assessment of Nuclear Energy Systems: Infrastructure, IAEA Nuclear Energy Series No. NG-T-3.12, IAEA, Vienna (2014).
- ↑ OECD NUCLEAR ENERGY AGENCY (NEA), Optimization of Geological Disposal of Radioactive Waste, OECD NEA report No. 6836, ISBN 978-92-64-99107-1, Paris (2010).
- ↑ 62.0 62.1 SWEDISH NUCLEAR FUEL AND WASTE MANAGEMENT CO. (SKB), Deep repository for spent nuclear fuel: SR 97 – Post-closure safety – Main report, Technical Report TR-99-06 (1999).
- ↑ 63.0 63.1 POSIVA, Plan for Safety Case of Spent Fuel Repository at Olkiluoto, Posiva Report 2005-01, Posiva, Finland, (2005).
- ↑ INTERNATIONAL ATOMIC ENERGY AGENCY, The Safety Case and Safety Assessment for the Disposal of Radioactive Waste, IAEA Safety Standards Series No. SSG-23, IAEA, Vienna (2012).
- ↑ OECD/NUCLEAR ENERGY AGENCY, Confidence in the Long-term Safety of Deep Geological Repositories: Its development and Communication, OECD Nuclear Energy Agency, Paris, (1999).
- ↑ OECD/NUCLEAR ENERGY AGENCY, Establishing and Communicating Confidence in the Safety of Deep Geologic Disposal, Approaches and Arguments, OECD Nuclear Energy Agency, Paris, (2002).
- ↑ OECD NUCLEAR ENERGY AGENCY (NEA), The post-closure radiological safety case for a spent fuel repository in Sweden, NEA/RWM/PEER(2012)2, NEA, Paris (2012).
- ↑ OECD NUCLEAR ENERGY AGENCY (NEA), Geological Disposal of Radioactive Waste: National Commitment, Local and Regional Involvement, OECD NEA report No. 7082, ISBN 978-92-64-99183-5, Paris (2012).
- ↑ INTERNATIONAL ATOMIC ENERGY AGENCY, INPRO Methodology for Sustainability Assessment of Nuclear Energy Systems: Economics, IAEA Nuclear Energy Series No. NG-T-4.4, IAEA, Vienna (2014).
- ↑ INTERNATIONAL ATOMIC ENERGY AGENCY, Methodology for the Assessment of Innovative Nuclear Reactors and Fuel Cycles, IAEA-TECDOC-1434, IAEA, Vienna (2004).
- ↑ 71.0 71.1 71.2 71.3 INTERNATIONAL ATOMIC ENERGY AGENCY, Estimation of Global Inventories of Radioactive Waste and Other Radioactive Materials, IAEA-TECDOC-1591, IAEA, Vienna (2007).
- ↑ 72.0 72.1 MASSACHUSETTS INSTITUTE OF TECHNOLOGY, The Future of Nuclear Power, An Interdisciplinary MIT Study, MIT, Boston (2003)
- ↑ 73.0 73.1 WORLD INFORMATION SERVICE ON ENERGY, Uranium Project, home page (2016), www.wise-uranium.org/nfcm.html .
- ↑ INTERNATIONAL PANEL ON FISSILE MATERIALS, Spent Nuclear Fuel Reprocessing in France, Research Report No.4. IPFM, Princeton (2008)