Important stressors in nuclear energy systems (Sustainability Assessment)
This page is the "Appendix I" to Environmental Impact of Stressors
Contents
- 1 Introduction
- 2 Mining of uranium ore
- 3 Milling of uranium ore
- 4 Refining and conversion of uranium ore to uranium hexafluoride
- 5 Enrichment of uranium
- 6 Conversion to uranium dioxide and fuel fabrication
- 7 Mixed oxide fuel fabrication
- 8 Power generation
- 9 Reprocessing
- 10 Storage of spent nuclear fuel away from the reactor
- 11 Disposal of radioactive waste
- 12 See also
- 13 References
Introduction
This appendix, including the tables, is based on Ref.[1]. It presents illustrative lists of important stressors
for facilities of an NES with a water cooled reactor using UOX or MOX fuel.
Important radionuclides, chemicals and other stressors which are relevant for various facilities of an NES
with a water cooled reactor using UOX or MOX fuel are presented in Sections 2 – 9, based on current experience.
However, it is recommended that, especially, in the case of an innovative design of an NES facility, this list should
be revised based on the actual radionuclide and chemical inventories in the innovative NES facility, their toxicity
and their environmental mobility.
In the case where calculation of the impact of stressors is necessary, it is desirable to maintain a conservative
approach, and to use internationally accepted models, such as those presented in Appendix II, or available licensed
national models. Model descriptions with appropriate references should be documented in the INPRO assessment
report.
The main facilities of an NES with a water cooled reactor using UOX and MOX fuel are as follows: mining
and milling of uranium ore, refining and conversion of uranium ore, enrichment of uranium, fabrication of nuclear
fuel, power generation, reprocessing of nuclear fuel, and radioactive waste storage and disposal including waste
from decommissioning. Some facilities of an NES have greater environmental impacts compared to others; all
facilities have specific stressors and impacts, and therefore each facility should be considered separately. Depending
on the scope of a specific Nuclear Energy System Assessment, only some of the NES facilities could be considered.
It should be mentioned that there are about 200 radionuclides which are specific to the different stages
of the nuclear fuel cycle and the reactor operation and which can cause radiation impacts on the environment.
In the following sections, only relatively long lived radionuclides are mentioned for each facility. However, it
should be recognized that short lived progenies, which are in equilibrium with some parent radionuclides, can
essentially contribute to the doses to public and biota species for many contamination scenarios. Some of these
short lived radionuclides are not discussed here explicitly; however, it is implied that their contributions to doses
have to be taken into account together with the impacts from their parent radionuclides in radiation impact analyses
(e.g. see Appendix II).
Mining of uranium ore
The environmental impacts of mining of uranium ores may involve the disruption of land surface and
water bodies, the release of radionuclides to air and water, the release of heavy metals and acids, the emission of
particulates, etc. In 2011, for example, conventional uranium mining produced 47% of the world’s uranium —
18% from open pits and 29% from underground mines[2]. Non-conventional methods have also been used
for uranium production, such as in situ leaching (ISL), which produced 42% of the world’s uranium in 2011.
The environmental impacts of a uranium mine are potentially numerous and diverse, depending on the mining
technologies used[3].
The environmental impacts of mining activities are measured using several criteria14. Individual dose is
normally used as the criterion for the radiological impact on humans and non-human species. Several other criteria
are used for the non-radiological environmental impact and for the radiological impact on the environment other
than humans.
Usually, baseline studies are conducted before any mining activities are undertaken; these studies are to be
used when establishing environmental quality objectives needed for the construction, operation, decommissioning
and postdecommissioning phases. In other cases, there are pre-established air, sediment, water and groundwater
quality objectives that need to be met. Social, aesthetic, economic and recreational values are met by establishing
requirements to preserve parts of the affected ecosystem, e.g. fish spawning areas and the habitats of endangered
species may need to be preserved for economic or social and ecological reasons.
Radionuclides of concern
Uranium ore contains natural occurring radioactive material (NORM), which includes uranium isotopes (mainly 238U) and uranium progenies (see Fig. 1) such as 230Th and 226Ra. The radioactive decay of the latter nuclides produces 222Rn (an inert gas), which is normally responsible for the biggest part of exposure to workers in the mines and often to the public.
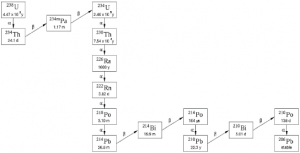
The important radionuclides which have to be considered in the environmental assessment are: 210Pb, 210Po,
226Ra, 222Rn, 228Th, 230Th, 232Th, 234Th, 234U, 235U and 238U. The environmental impact from 227Ac (a decay product
of 235U) is also recommended to be studied. As mentioned above, short lived progenies such as 214Bi and 214Pb
(both progenies of 222Rn, see Fig. 1) are responsible for a large portion of the radiation impact, but are not listed
here because they are always in equilibrium with 222Rn.
For the majority of these radionuclides (except for 222Rn), contamination of water with radionuclides is the
most important exposure pathway. The radioactivity of water is generally stipulated by the dissolved uranium,
thorium, radium and lead ions. However, an aerial pathway can be also important for several radionuclides,
e.g. 226Ra, 230Th and 238U.
Some natural radionuclides, e.g. uranium, provide both radiation and chemical environmental impacts, which
also have to be taken into account in the assessment.
Chemicals of concern
Water contaminated with chemicals (including NORM) is produced by dewatering of underground and open pit mines, surface water runoff from and seepage through the waste rock piles and ore stockpiles, and ISL restoration activities. In situations when the ore includes pyrites, the generation of acid requires neutralization as part of the water treatment process, if this water is planned to be discharged to the environment. Acid generation is a concern of all types of mining because the acid solubilizes and increases the mobility of heavy metals, and for uranium mining, it also mobilizes radionuclides. Utilization of explosives in mining may add some nitrites, nitrates and ammonia to the mine wastewater.
Other stressors
Examples of other stressors are:
- Temporarily committed land, e.g. for a current NES (using an open fuel cycle with light water reactors (LWRs)), this value is estimated to be about 25 ha/GW(e) for open pit mining[1];
- Permanently committed land;
- Dust, originating at exposed ore stockpiles, ore haul roads, etc.
Illustrative list of stressors
An illustrative list of stressors for evaluation of the environmental impact from uranium ore mining is presented in Table 1.
Stressor | Media type | Normalized release units | Average release units |
---|---|---|---|
Radionuclides | |||
Pb-210 | Water* | MBq/tU or MBq/GW·a | MBq/a or MBq/d |
Po-210 | Water | ||
Ra-226 | Air, water | ||
Ra-228 | Air, water | ||
Th-228 | Air, water | ||
Th-230 | Air, water | ||
Th-232 | Air, water | ||
Th-234 | Air, water | ||
U-234 | Air, water | ||
U-235 | Air, water | ||
U-238 | Air, water | ||
Rn-222 | Air | ||
Ac-227 | Air, water | ||
Chemicals | |||
As | Water | kg/tU or kg/GW·a | kg/a or kg/d |
Se | Water | ||
Ni | Water | ||
Nirites | Water | ||
Nitrates | Water | ||
Ammonia | Water | ||
Sulphates | Water | ||
Others | |||
Land temporarily committed | Soil | m2/tU or m2/GW | m2 (total area) |
Land permanently committed | Soil | ||
Dust | Air | kg/tU | kg/a |
Solids dissolved | Water | kg/tU | kg/a |
Solids suspended | Water | kg/tU | kg/a |
* Water comprises surface water as well as groundwater
Milling of uranium ore
Usually, uranium ore is processed close to the mine to limit transportation costs. The typical process for the
extraction of uranium consists of crushing and grinding of the ore, followed by chemical leaching with sulphuric
acid or an alkali carbonate solution. Acid leaching is the more common method; however, some mills use alkaline
leaching when the ore body contains limestone or similar basic constituents, which would consume uneconomic
quantities of acid. The uranium solution is purified and concentrated by ion exchange and/or solvent extraction
technology. The uranium is then precipitated from solution, filtered and dried to produce a concentrate, known as
yellowcake, which contains between 60% and 90% uranium by weight.
Potential sources of impacts on the environment from mill tailings comprise the following types of release:
(i) escape of gaseous radon; (ii) transport of radioactive particulates by the wind; (iii) contamination of surface
water and groundwater through runoff or seepage of radionuclides, heavy metals or other toxic materials; and
(iv) dispersion of tailings over a wide area caused by erosion or flooding.
Radionuclides of concern
At the stage of milling, radionuclides of concern are similar to those which are considered for mining. The tailing slurry is the most significant source of radionuclides. Mill sites in dry areas give rise to effectively no liquid effluents. However, the runoff water from mills in wet climates will contain radionuclides and may need treatment before release into watercourses. The tailings are characterized by their relatively large volumes and relatively low activity concentrations of long lived natural occurring radionuclides. About 15% of the total radioactivity which was originally contained in the ore is retained in the yellowcake produced by the mill. Once shorter lived radioactive nuclides have decayed, some 70% of the radioactivity originally present in the ore is left in the tailings. The tailings contain nearly all of the naturally occurring radioactive progenies from the decay of uranium, notably 226Ra and 230Th. The presence of 230Th provides a long term source of radon emission. Radioactive airborne effluents from milling may include dusts and radon gas released into the air from ore stockpiles, crushing and grinding of ore, drying and packing of yellowcake, and the tailings retention system. The releases of dusts produced in the processing operations are reduced by ventilation extract scrubbers. Tailings may be a continuing source of radon and radioactive dust after milling operations have ceased. Thus, the list of radionuclides that have to be evaluated for milling is similar to the one for mining.
Chemicals of concern
Contaminated water is discharged from uranium mills to tailing management facilities. The contaminants
include heavy metals, sulphates, chlorides, organics and ammonia. The exact mixture will depend on such factors
as type of process used and ore grade, and should be evaluated separately for each case.
Airborne chemical contaminants released to the environment include combustion products (oxides of
carbon, nitrogen and sulphur) from the process steam boilers and power generation, sulphuric acid fumes in small
concentrations from the leach tanks and vaporized organic reagents from the solvent extraction ventilation system.
In addition, from some plants where sulphuric acid is made on-site, sulphur dioxide is released to the atmosphere.
Other stressors
It is estimated that currently an average of about 4 ha of land area is required for milling of uranium ore sufficient to produce 1 GW(e) of energy in a once through fuel cycle. About 75% of this land is devoted to impoundment for the permanent disposal of mill tailings. The amount of land required is highly dependent on the grade of ore being processed. Lower grade ore milling operations normally use more land per GW(e) compared with facilities processing higher grade ore.
Illustrative list of stressors
An illustrative list of stressors for evaluation of the environmental impact from uranium ore milling is presented in Table 2.
Stressor | Media type | Normalized release units | Average release units |
---|---|---|---|
Radionuclides | |||
Pb-210 | Water | MBq/tU or MBq/GW·a | MBq/a or MBq/d |
Po-210 | Water | ||
Ra-226 | Air, water | ||
Th-228 | Water | ||
Th-230 | Air, water | ||
Th-232 | Water | ||
Th-234 | Water | ||
U-234 | Water | ||
U-235 | Water | ||
U-238 | Water | ||
Rn-222 | Air | ||
Ac-227 | Water | ||
Dust (total alpha activity) | Air | ||
Chemicals | |||
Heavy metals | Water | kg/tU or kg/GW·a | kg/a or kg/d |
Organic | Water | ||
Chlorides | Water | ||
Ammonia | Water | ||
Sulphates | Air, water | ||
Others | |||
Land temporarily committed | Soil | m2/tU | m2 (total area) |
Land permanently committed | Soil | ||
Dust | Air | kg/tU | kg/a |
Solids dissolved | Water | kg/tU | kg/a |
Solids suspended | Water | kg/tU | kg/a |
Refining and conversion of uranium ore to uranium hexafluoride
Uranium ore concentrate (UOC) may consist of any of several different uranium compounds. It still contains elements other than uranium and some uranium radioactive decay products. UOC is refined to obtain higher purity uranium compounds. Two different processes — wet and dry — are used to purify UOC and convert it into a usable form. The more commonly used process, the wet process, consists of dissolving UOC in nitric acid and refining it by solvent extraction. The pure uranyl nitrate formed is then converted to uranium trioxide, which can be converted to uranium dioxide (UO2) for natural uranium fuel, or converted progressively to uranium tetrafluoride and uranium hexafluoride (UF6) for enrichment to produce LWR fuel
Radionuclides of concern
Emissions from uranium refining and conversion facilities are relatively minor. The only radioactive emission
of significance is natural uranium, which may be emitted as dust or as a volatile reaction product of UF6[1]. The
plants are equipped with dust collectors and scrubbers to mitigate the impacts of these emissions.
The effluents from the wet and dry processes of UF6 production differ substantially. In the wet process, most
of the impurities entering with UOC are rejected in the raffinate solution from solvent extraction, whereas in the
dry process, most of the UOC impurities are contained in solid wastes from the fluorination and distillation stages.
In the case when raffinate from the wet process is recycled through the uranium mill where residual uranium
is recovered and the remaining waste is disposed of with the tailings, the radiological impact of the raffinate is
included in the radiological impact of the milling facility. Where the raffinate is not recycled, the radiological
impact is due to residual thorium and, to a lesser extent, radium.
Chemicals of concern
Atmospheric emissions from the refining and conversion processes contain greenhouse gases such as NOX. Aqueous release may contain residual tributylphosphate and heavy metals that could have a potential impact on benthic fauna. Other contaminants with potential for environmental impacts are nitrates, and, under accident conditions, ammonia and fluorides may also be released.
Other stressors
Of the temporary land commitment for a UF6 production plant (~1.3 ha/GW(e)), approximately 10% is needed for roads, fills and plant structures[1]. Approximately 1% of land needed for a plant is permanently committed for waste burial.
Illustrative list of stressors
An illustrative list of stressors for evaluation of the environmental impact from refining and conversion is presented in Table 3.
Stressor | Media type | Normalized release units | Average release units |
---|---|---|---|
Radionuclides | |||
Pb-210 | Water | MBq/tU or MBq/GW·a | MBq/a or MBq/d |
Po-210 | Water | ||
Ra-226 | Air, water | ||
Th-228 | Air, water | ||
Th-230 | Air | ||
Th-232 | Air | ||
Th-234 | Air, water | ||
U-234 | Water | ||
U-235 | Air, water | ||
U-238 | Air, water | ||
Chemicals | |||
Heavy metals (have to be specified within the assessment) | Water | kg/tU or kg/GW·a | kg/a or kg/d |
Fluorides | Air, water | ||
Nitrogen oxides | Air | ||
Sulphur oxides | Air | ||
Carbon monoxide | Air | ||
Volatile organic compounds | Air | ||
Tributylphosphate | Water | ||
Others | |||
Land temporarily committed | Soil | m2/tU or m2/GW | m2 (total area) |
Land permanently committed | Soil | ||
Heat rejected | Air | MW(th)/tU | MW(th)/a |
Solids dissolved | Water | kg/tU | kg/a |
Solids suspended | Water | kg/tU | kg/a |
Enrichment of uranium
LWRs normally use uranium enriched to about 4–5% in 235U. Current commercial enrichment technologies are based on gaseous diffusion or centrifugation of uranium isotopes in the form of UF6. Reference[5] briefly explains the gas diffusion process as follows:
“In the gaseous diffusion process, gaseous UF6 is compressed and passed over a porous membrane. Molecules of UF6 containing the lighter isotope, uranium-235, diffuse through the membrane more rapidly than those with the heavier uranium-238 isotope; consequently, the UF6 passing the membrane has a slightly greater proportion of molecules containing uranium-235. The degree of enrichment for one membrane is minute and over a thousand successive diffusion stages are necessary to raise the proportion of uranium-235 from the naturally occurring level of 0.71 per cent to the 2–4 per cent required in the product stream, with about 0.25 per cent in a reject ‘tails’ stream.
“Each stage requires recompression of the gaseous hexafluoride. Uranium enrichment by gaseous diffusion requires large quantities of electrical energy. About 36.5 MWe-y of electricity would be needed by a gaseous diffusion plant to enrich the uranium for the generation of 1 GWe of electricity in a LWR”.
For the the uranium enrichment by centrifugation process, Ref.[5] provides the following simplified description:
“During enrichment by gas centrifugation, molecules of UF6 containing the heavier isotope uranium-238 migrate preferentially to the wall of a rapidly rotating cylinder. There is a consequent enrichment in the lighter uranium-235 isotope in the gas near the tube axis. The separation factor is greater than in the diffusion process, and the two streams removed from the tube axis and wall require only tens of stages arranged in a cascade to produce the required percentages of uranium-235 in the product and the reject tails. High centrifugal stresses limit the size of the equipment, and many parallel cascades involving hundreds of thousands of centrifuges are required to achieve the separative capacity of a commercial enrichment plant.”
Radionuclides of concern
Emissions of radionuclides from the enrichment process are generally small and consist essentially of long lived uranium isotopes, such as 234U, 235U and 238U, together with 234mPa and 230Th, which are the short lived decay products of 238U. The long half-life of 230Th prevents activity buildup of any other radionuclide of the 238U series. For current facilities, the radiological impacts on the public and non-human species are negligible. At the Capenhurst enrichment site in the United Kingdom, doses to the critical group members of the public are estimated to be less than 0.01 mSv per year.
Chemicals of concern
The enrichment plant itself generates small quantities of airborne fluorides and oxides of nitrogen and sulphur from the process cooling systems, process cleanup operations, on-site steam plant and auxiliary production facilities. Uranium losses in the effluents are very low. Some sludge from container cleanup operations is usually retained on-site. The depleted uranium residue from enrichment plants is normally stockpiled for possible future recovery of the remaining fissile material. The depleted uranium can be used in the future as a resource, and is therefore not considered as a release (or waste). It is noted that near current enrichment facilities, the concentrations of gaseous and liquid effluents are below the ranges for which deleterious effects have been observed.
Other stressors
Essentially, none of the land required for enrichment facilities is committed permanently. The temporary land requirement is estimated to be 0.3 ha/GW(e). Water is required for the operation of cooling towers associated with the gaseous diffusion process and also for the large amounts of electricity that need to be generated. For enrichment by gaseous diffusion, 92% of all the electricity required in a nuclear fuel cycle up to fuel fabrication is consumed in enrichment. Other enrichment techniques such as centrifuges require less than one tenth of the electricity needed for a gaseous diffusion plant.
Illustrative list of stressors
An illustrative list of stressors for evaluation of the environmental impact from an enrichment plant is presented in Table 4.
Stressor | Media type | Normalized release units | Average release units |
---|---|---|---|
Radionuclides | |||
Ra-226 | Water | MBq/tU or MBq/GW·a | MBq/a or MBq/d |
Rn-222 | Air | ||
Tc-99 | Air, water | ||
Th-234 | Air | ||
U-234 | Air, water | ||
U-235 | Air, water | ||
U-238 | Air, water | ||
Chemicals | |||
Fluorides | Air, water | kg/tU or kg/GW·a | kg/a or kg/d |
Nitrogen oxides | Air | ||
Sulphur oxides | Air | ||
Carbon monoxide | Air | ||
Volatile organic compounds | Air | ||
Tributylphosphate | Water | ||
Others | |||
Land temporarily committed | Soil | m2/tU or m2/GW | m2 (total area) |
Land permanently committed | Soil | ||
Dust | Air | kg/tU | kg/a |
Dust (radioactive) | Air | MBq/tU | MBq/a |
Solids dissolved | Water | kg/tU | kg/a |
Solids suspended | Water | kg/tU | kg/a |
Conversion to uranium dioxide and fuel fabrication
The required material for the fabrication of fuel for an LWR is UO2. The UF6 (enriched to about 4–5% in 235U) is converted to UO2 powder, which is formed into pellets, sintered to achieve the desired density and ground to the required dimensions. Fuel pellets are loaded into tubes of zirconium alloy (zirconium–tin or zirconium–niobium), which are sealed at both ends. These fuel rods are spaced in fixed parallel arrays to form reactor fuel assemblies.
Radionuclides of concern
The radionuclides of concern are about the same as those described for enrichment. The most important pathway of the population and non-human species exposure is inhalation. The doses due to liquid discharges are much less than those from airborne discharges.
Chemicals of concern
The effluent from fuel fabrication with the greatest potential environmental impact is chemical in nature.
Hydrogen fluoride (HF) is potentially the most significant airborne chemical effluent from fuel fabrication.
Liquid effluent from fuel manufacture contains nitrogen compounds formed from ammonia in the production of
UO2 powder and by nitric acid in the scrap recovery operations. Very small quantities of uranium are released with
the effluent gases and liquids. Ammonia and nitrates are found in liquids released from the waste holding ponds.
In a dry fuel production process, the emissions of HF to the environment are very small. Nearly all of the
HF produced will be removed from the off-gas by the condensing and cleaning system. The end products, liquid HF
and CaCO3/CaF2, are suitable for industrial use. Thus, the non-radiological impacts are mitigated by the recovery
and recycling of potential contaminants.
Other stressors
The temporary land requirement is estimated to be 0.1 ha/GW(e) for an NES with an LWR reactor. All of the land required for fuel fabrication can be reclaimed by conventional techniques. Care would have to be taken when decommissioning the holding ponds or lagoons. The facility requires water, most of which is used for cooling of plant processes. The water does not come into contact with uranium or process chemicals during operation. In the dry process, there is no cooling water discharge.
Illustrative list of stressors
An illustrative list of stressors for evaluation of the environmental impact from UO2 fuel fabrication is presented in Table 5.
Stressor | Media type | Normalized release units | Average release units |
---|---|---|---|
Radionuclides | |||
Ra-226 | Air | MBq/tU or MBq/GW·a | MBq/a or MBq/d |
Th-234 | Air | ||
U-234 | Air, water | ||
U-235 | Air, water | ||
U-238 | Air, water | ||
Chemicals | |||
Fluorides | Air, water | kg/tU or kg/GW·a | kg/a or kg/d |
Nitrogen oxides | Air | ||
Ammonia | Water | ||
Others | |||
Land temporarily committed | Soil | m2/tU or m2/GW | m2 (total area) |
Land permanently committed | Soil |
Mixed oxide fuel fabrication
Two processes are currently used to produce MOX fuel; these processes differ mainly at the beginning,
owing to the nature of their feed materials. For the dry process, the feed materials are UO2 (ammonium uranyl
carbonate, ammonium diuranate and integrated dry route) and PuO2 powders. The alternative wet process starts
with plutonium and uranium nitrate solutions. MOX powder is prepared by co-milling or co-conversion, depending
on the type of feed material.
Following the main fabrication, the steps (pelletizing, sintering, rod fabrication and assembling) are
comparable with those of uranium fuel fabrication. The main difference to UO2 fuel fabrication is related to a strict
alpha activity containment of the processed material in tight gloveboxes and shielding against gamma and neutron
radiation.
Radionuclides of concern
Atmospheric discharges result from ventilation — for dynamic containment purposes — of production
buildings and gloveboxes wherein the manufacturing process is performed. The discharges consist of aerosols of
uranium/plutonium.
Very low alpha active liquids, principally from cleaning of non-contaminated or very low contaminated areas
(operators, floors, etc.), are discharged into the environment after nuclear control measurements (range of activity:
1–5 kBq/m3). The balance of discharged activity is exactly maintained.
Liquid discharges increase with the throughput of a plant (these discharges mainly depend on the number of
workers and the surface of the buildings); typical discharged activities are in the range 0.5–3 MBq/a.
Chemicals of concern
The chemical emissions from this process are negligible (for the dry process, there are no chemical emissions).
Other stressors
Basically, MOX fuel fabrication is a recycling activity. Plutonium is a valuable fissile material originating from the reprocessing of UO2 spent fuel. The replacing of 235U by plutonium in LWR fuel contributes to a more efficient use of uranium resources. In most cases, for the fuel matrix, natural uranium, depleted uranium and reprocessed uranium can be used. Utilization of other resources (e.g. energy, fluids and land) is comparable with those used in UO2 fuel fabrication. The land requirement is estimated to be 3 ha/GW(e). The dry process does not use water, and its energy requirement is estimated to be 0.3 MW/a per GW(e).
Illustrative list of stressors
An illustrative list of stressors for evaluation of the environmental impact from MOX fuel fabrication is presented in Table 6.
Stressor | Media type | Normalized release units | Average release units |
---|---|---|---|
Radionuclides | |||
Pu | Air | MBq/tU or MBq/GW·a | MBq/a or MBq/d |
U-234 | Air, water | ||
U-235 | Air, water | ||
U-238 | Air, water | ||
Others | |||
Land temporarily committed | Soil | m2/tU or m2/GW | m2 (total area) |
Land permanently committed | Soil |
Power generation
Radionuclides of concern
Discharges from nuclear reactors (LWRs and heavy water reactors) to the atmosphere consist mainly of the
noble (inert) gases: 41Ar, 85Kr, 85mKr, 88Kr, 131Xe, 133Xe, 133mXe, 135Xe and 138Xe. Most of these radionuclides, except
for 85Kr with a half-life of 10.8 years, are short lived, and their environmental impacts are essentially limited. Other
radioactive gases include 14C, 3H and 131I.
Radionuclides in liquid discharges with potential environmental impacts consist of 134Cs, 137Cs, 51Cr, 58Co,
3H, 55Fe, 54Mn, 110mAg and 65Zn.
Examples of the most important radionuclides that can be discharged from nuclear power plants are briefly
discussed in Ref.[6]. A UK study[7] provides detailed information on discharges of radionuclides from two
water cooled reactors of latest design, namely the European pressurized reactor (EPR) (AREVA) and the AP1000
(Westinghouse) reactor. Data on estimation of releases of radionuclides from the AP1000 reactor, the EPR, the
economic simplified boiling water reactor (ESBWR) (GE Hitachi) and the ACR-1000 reactor (Atomic Energy of
Canada Limited), and operational data for their predecessor designs, are summarized in Ref.[8].
Chemicals of concern
Atmospheric emissions of chemicals from this process are negligible. Aqueous releases may contain sewage with organic compounds and heavy metals originating from auxiliary facilities, such as radioactive waste facilities, the condensate polishing plant, the steam generator blowdown system, the laundry, laboratories, etc. The Environment Agency provides comprehensive information on discharges of chemicals from the EPR and the AP1000 reactor in Ref.[9].
Other stressors
Other stressors are: temporary land requirement estimated to be about 50 ha for a 1.2 GW(e) nuclear power plant[10]; use and depletion of water resources and disturbance of the hydrological state of surface water and groundwater; heat discharge; and changes in the biodiversity of non-human species in the terrestrial and aquatic ecosystems in the vicinity of a facility.
Illustrative list of stressors
An illustrative list of stressors for evaluation of the environmental impact from power generation is presented in Table 7.
Stressor | Media type | Normalized release units | Average release units |
---|---|---|---|
Radionuclides | |||
H-3 | Air, water | MBq/tU or MBq/GW·a | MBq/a or MBq/d |
C-14 | Air, water | ||
Na-24 | Water | ||
Ar-41 | Air | ||
Cr-51 | Air, water | ||
Mn-54 | Water | ||
Fe-55 | Water | ||
Co-58 | Air, water | ||
Fe-59 | Water | ||
Co-60 | Air, water | ||
Ni-63 | Water | ||
Zn-65 | Water | ||
Kr-85 | Air | ||
Kr-85m | Air | ||
Kr-87 | Air | ||
Kr-88 | Air | ||
Sr-89 | Air, water | ||
Sr-90 | Air, water | ||
Y-91 | Water | ||
Zr-95 | Air, water | ||
Nb-95 | Air, water | ||
Tc-99m | Water | ||
Ru-103 | Air, water | ||
Ag-110m | Air, water | ||
Te-123 | Water | ||
Sb-124 | Water | ||
Sb-125 | Water | ||
Xe-131m | Air | ||
I-131 | Air | ||
I-133 | Air, water | ||
Xe-133 | Air | ||
Xe-133m | Air | ||
Cs-134 | Air, water | ||
Xe-135 | Air | ||
Xe-135m | Air | ||
Cs-136 | Water | ||
Cs-137 | Air, water | ||
Xe-137 | Air | ||
Xe-138 | Air | ||
Ba-140 | Air, water | ||
La-140 | Water | ||
Ce-144 | Air, water | ||
Pr-144 | Water | ||
Pu-241 | Water | ||
Chemicals | |||
Organic compound | Water | kg/tU or kg/GW·a | kg/a or kg/d |
Heavy metals | Air, water | ||
Other chemicals[9] | Air, water | ||
Others | |||
Land temporarily committed | Soil | m2/tU or m2/GW | m2 (total area) |
Land permanently committed | Soil | ||
Heat rejected | Air, water | MW(th)/tU or MW(th)/GW·a | MW(th)/a |
Water quality solids dissolved | Water | kg/tU or kg/GW·a | kg/a |
Direct damage solids suspended | Water |
Reprocessing
The principal objectives of reprocessing are: (i) to recover uranium and plutonium for reuse as nuclear fuels;
(ii) to separate radioactive and neutron absorbing fission products; and (iii) to convert the radioactive wastes of
spent fuel reprocessing into forms that are suitable for safe storage and disposal. The difference between a once
through fuel cycle of an NES and a reprocessing cycle (also called a partly closed fuel cycle) is that the reprocessing
cycle makes more efficient use of the fuel through extraction of plutonium and recycling of the residual 235U.
The spent fuel is first stored to allow its thermal power and its radioactivity to decrease to the design levels
of the reprocessing plant. Generally, for spent LWR fuels, the total cooling time is at least three years. The fuel
cooling pond water can become slightly contaminated, primarily with 137Cs, 60Co, 54Mn and 90Sr, and needs to
be decontaminated by filtration, to remove particulates, and by ion exchange, to absorb the activity present in a
soluble form.
In the following, the currently used industrial (wet) process for reprocessing of spent uranium fuel (plutonium
uranium redox extraction — PUREX) is described. The spent fuel is mechanically chopped into small pieces,
which are dipped into a dissolver solution. The fuel is leached from the chopped cladding by boiling nitric acid.
After leaching, the fuel hulls (chopped cladding) are washed and transferred to a storage. The liquid in the dissolver
will contain some insoluble material, including fission product compounds from the spent fuel and both coarse
and fine particulates from the cladding material, together with debris from the structural components of the fuel
elements. These insoluble residues are separated by centrifugation or filtration. Both these wastes will need to
be stored prior to disposal. The clear solution is analysed to determine its nuclear fuel content and is eventually
adjusted for uranium–plutonium concentration and acidity prior to extraction.
The liquid from the dissolver, after the removal of solids, is passed to successive extraction systems to
separate the fission product wastes from the uranium and plutonium. The products from the solvent extraction
cycles are uranium and plutonium nitrates, while the bulk of the fission products are removed as high level wastes
for storage prior to conditioning and ultimate disposal. The solvent of the washing process is reused. The two
products of the solvent extraction process are first concentrated by evaporation. The uranyl nitrate solution can then
be converted to UOX form. Subsequently, this product can be sent to another site for conversion to UF6 and used in
a re-enrichment cycle. The plutonium nitrate is converted first to plutonium oxalate by a precipitation process and
subsequently to the oxide by thermal decomposition and calcination.
Radionuclides of concern
Reprocessing is one of the most important sources of radionuclide release to the environment. The radionuclides of particular interest in airborne discharges include 137Cs, 14C, 3H and 129I, and in liquid discharges 137Cs, 14C, 3H, 129I, 106Ru and 90Sr. Additionally, reprocessing of nuclear fuel results in the discharge of actinides such as 241Am, 237Np, 239Pu, 240Pu and 241Pu.
Chemicals of concern
Releases of chemicals from reprocessing facilities are negligible. For example, Ref.[1] gives an estimation of discharge of non-radiological liquid effluent from the Thorp reprocessing plant in the United Kingdom on the level that produces pollutant concentrations that are less than 1% of the relevant environmental quality standards.
Other stressors
The temporary land requirement is estimated to be 1.5 ha/GW(e). About 95% of this land use is a fenced-in buffer zone and is not disturbed. The La Hague (France) reprocessing site is a 300 ha complex and its reprocessing capacity is 1600 t/a. It also has 14 400 t of spent fuel storage pools capacity.
Illustrative list of stressors
An illustrative list of stressors for evaluation of the environmental impact from wet reprocessing of nuclear fuel is presented in Table 8.
Stressor | Media type | Normalized release units | Average release units |
---|---|---|---|
Radionuclides | |||
H-3 | Air, water | MBq/tU or MBq/GW·a | MBq/a or MBq/d |
C-14 | Air, water | ||
Na-24 | Water | ||
P-32 | Air, water | ||
Sc-46 | Air, water | ||
Si-41 | Water | ||
Cr-51 | Water | ||
Fe-55 | Air, water | ||
Co-58 | Air, water | ||
Mn-54, 56, 58 | Air, water | ||
Co-60 | Air, water | ||
Cu-64 | Water | ||
Zn-65 | Air, water | ||
As-76 | Air | ||
Kr-85 | Air | ||
Kr-85m | Air | ||
Kr-87 | Air | ||
Zr-95 | Air, water | ||
Nb-95 | Air, water | ||
Sr-90 | Air, water | ||
Ru-106 | Air, water | ||
Sb-124 | Water | ||
I-129 | Air, water | ||
I-131 | Air, water | ||
Xe-133 | Air | ||
Xe-133m | Air | ||
Xe-135 | Air | ||
Xe-138 | Air | ||
Cs-134 | Air | ||
Cs-137 | Air, water | ||
Ba-140 | Air, water | ||
La-140 | Air, water | ||
Ce-141 | Air, water | ||
Ce-144 | Air, water | ||
Eu-152 | Water | ||
Eu-154 | Water | ||
Pu-239 | Air, water | ||
Pu-240 | Air, water | ||
Pu-241 | Air, water | ||
Np-237 | Air, water | ||
Am-241 | Air, water | ||
Chemicals | |||
Organic compound | Water | kg/tU or kg/GW·a | kg/a or kg/d |
Heavy metals | Air, water | ||
Others | |||
Land temporarily committed | Soil | m2/tU or m2/GW | m2 (total area) |
Land permanently committed | Soil |
Storage of spent nuclear fuel away from the reactor
Compared to other nuclear facilities of an NES, rather minor stressors occur in facilities away from reactors for storage of spent nuclear fuel. No chemicals are expected to be released from such a facility. Spent fuel can be stored in dry casks or in fuel ponds. Environmental stressors from a dry storage and a wet storage essentially differ. A preliminary list of stressors for the evaluation of the environmental impact from a wet storage facility is presented in Table 9.
Stressor | Media type | Normalized release units | Average release units |
---|---|---|---|
Radionuclides | |||
Co-60 | Air | MBq/tU or MBq/GW·a | MBq/a or MBq/d |
Cs-137 | Air | ||
Others | |||
Land temporarily committed | Soil | m2/tU or m2/GW | m2 (total area) |
Land permanently committed | Soil |
Disposal of radioactive waste
Releases from radioactive waste disposal facilities including facilities for direct disposal of spent nuclear fuel are discussed in a separate manual of the INPRO methodology covering the NES sustainability assessment in the area of waste management. In this current INPRO Manual on the environmental impact of stressors, the waste disposal facilities are considered in UR2 along with other NES installations contributing to the total radiotoxicity, and in UR3, which is focused on the optimization of measures to reduce environmental impacts.
See also
[ + ] Assessment Methodology | |||||
---|---|---|---|---|---|
|
References
- ↑ 1.0 1.1 1.2 1.3 1.4 INTERNATIONAL ATOMIC ENERGY AGENCY, Health and Environmental Aspects of Nuclear Fuel Cycle Facilities, IAEA-TECDOC-918, IAEA, Vienna (1996).
- ↑ OECD NUCLEAR ENERGY AGENCY, INTERNATIONAL ATOMIC ENERGY AGENCY, Uranium 2011: Resources, Production and Demand, OECD, Paris (2012).
- ↑ INTERNATIONAL ATOMIC ENERGY AGENCY, Best Practice in Environmental Management of Uranium Mining, IAEA Nuclear Energy Series No. NF-T-1.2. IAEA, Vienna (2010).
- ↑ INTERNATIONAL ATOMIC ENERGY AGENCY, Guidance for the Application of an Assessment Methodology for Innovative Nuclear Energy Systems, INPRO Manual: Environment, Vol. 7, Final Report of Phase 1 of the International Project on Innovative Nuclear Reactors and Fuel Cycles (INPRO), IAEA-TECDOC-1575/Rev. 1, IAEA, Vienna (2008).
- ↑ 5.0 5.1 COSTELLO, J.M., Nuclear Fuel Manufacture (Symp. Nuclear Technology, Institution of Mechanical Engineers, Melbourne, 1980).
- ↑ INTERNATIONAL ATOMIC ENERGY AGENCY, Managing Environmental Impact Assessment for Construction and Operation in New Nuclear Power Programmes, IAEA Nuclear Energy Series No. NG-T-3.11, IAEA, Vienna (2014).
- ↑ 7.0 7.1 JONES, A., JONES, K., HOLMES, S., EWERS, L., CABIANCA, T., Assessing the possible radiological impact of routine radiological discharges from proposed nuclear power stations in England and Wales, J. Radiol. Prot. 33 1 (2013) 163.
- ↑ ENVIRONMENT AGENCY, Study of Historical Nuclear Reactor Discharge Data, Science Rep. SC070015/SR1, Environment Agency, Bristol (2009).
- ↑ 9.0 9.1 9.2 ENVIRONMENT AGENCY, Chemical Discharges from Nuclear Power Stations: Historical Releases and Implications for Best Available Techniques, Science Rep. SC090012/R1, Environment Agency, Bristol (2011).
- ↑ FENNOVOIMA OY, Environmental Impact Assessment Report for a Nuclear Power Plant, Fennovoima Oy, Helsinki (2014).