Environmental Impact from Depletion of Resources (Sustainability Assessment)
INPRO basic principle (BP) in the area of environmental impact by depletion of resources – The NES shall be capable of contributing to the energy needs in the twenty-first century while making efficient use of non-renewable resources.
Contents
- 1 Introduction
- 2 General features and necessary INPUT for an assessment
- 3 INPRO Basic Principle, User Requirement and Criteria
- 3.1 INPRO Basic Principle: availability of resources
- 3.2 User requirement UR1: consistency with resource availability
- 3.2.1 Criterion CR1.1: Fissile/fertile material
- 3.2.2 Criterion CR1.2: Non-renewable materials
- 3.2.3 Criterion CR1.3: Power supply to the nuclear energy system
- 3.2.4 Criterion CR1.4: End use of uranium
- 3.2.5 Criterion CR1.5: End use of thorium
- 3.2.6 Criterion CR1.6: End use of other non-renewable resources
- 3.3 User requirement UR2: adequate net energy output
- 4 Appendixes
- 5 References
Introduction
Objective
This volume of the updated INPRO manual provides guidance to the assessor of an NES (or a facility thereof)
that is to be installed, describing how to apply the INPRO methodology in the area of environmental impact from
depletion of resources. The INPRO assessment should enable either the confirmation of adequate environmental
performance by the NES, i.e. fulfilment of all INPRO methodology environmental CR, or the identification of gaps
(non-compliance with the INPRO methodology CR) requiring corrective actions (including research, development
and demonstration (RD&D)) to achieve long term sustainability of the NES assessed.
The INPRO assessor (or team of assessors) is assumed to be knowledgeable in the area of environmental
impact from depletion of resources and/or may be using the support of qualified national or international
organizations (e.g. the IAEA) with relevant experience.
Two general types of INPRO assessors can be distinguished: a designer (supplier or developer) of nuclear
technology, i.e. a nuclear technology holder, and a (potential) user of such technology.
The role of the latter type of assessor, i.e. a technology user, is primarily to check, in a simple manner, whether
the designer (supplier) has appropriately taken into account the resource aspects in their design as defined by the
INPRO methodology. A technology user is assumed, in order to minimize their risk, to be primarily interested in
proven technology to be installed in their country.
A designer (developer) performing an INPRO assessment can also use this current publication to check
whether the (innovative) design under development meets the INPRO methodology requirements, but can
additionally initiate modifications during early design stages, if necessary, to improve the performance of the
design.
An assessor in a country embarking on a nuclear power programme could use the INPRO methodology in a
so called graded approach, depending on the stage of the programme (see overview of the INPRO methodology).
Guidance provided here, describing good practices, represents expert opinion but does not constitute
recommendations made on the basis of a consensus of Member States.
Scope
Environmental impact from an NES involves two large groups of factors. The first group comprises
radiological, chemical, thermal and other stressors which NESs release into environment. This group also includes
water intake because this factor can be important for biota even when this water is returned to the environment in
a clean form (e.g. as steam from NPP cooling towers). All these factors are considered in the INPRO methodology
manual on environmental impact of stressors[1].
The second group of factors impacting the environment comprises the consumption of non-renewable resources
including both fissile/fertile materials necessary to produce nuclear fuel and other materials (e.g. zirconium). All
these factors and consumption of electricity necessary to construct, operate and occasionally decommission NES
installations are considered in this updated INPRO methodology manual on environmental impact from depletion
of resources.
Structure
In Section 2, general features of an environmental assessment on the impact of depletion of resources and an
overview of information that must be available to an INPRO assessor to perform assessment are presented.
In Section 3, the background of the INPRO methodology BP for environmental impact from depletion of
resources, and the corresponding URs and CR, consisting of INs and ALs, are presented. At the CR level, guidance
is provided on how to determine the value of the IN and AL.
Appendix I summarizes information on global demand and supply of fissile and fertile materials.
Appendix II presents information on global demand and supply of non-renewable materials (other than fissile
and fertile materials).
Appendix III summarizes relevant results of the INPRO collaborative project on Global Architectures of
Innovative Nuclear Energy Systems with Thermal and Fast Reactors, Including a Closed Fuel Cycle (GAINS)[2],
and provides references to some other studies that reflect upon uranium supply and demand in particular global
NES evolution scenarios[3].
Appendix IV presents the results of an evaluation of the mass balance of an NES focusing on the front end
of the fuel cycle. The tool used for the evaluation is the NESA Economic Support Tool (NEST) described in the
INPRO methodology manual on economics[4] (NESA stands for nuclear energy system assessment).
Table 1 provides an overview of the BP, URs and CR in the INPRO methodology area of environmental
impact from depletion of resources
INPRO basic principle the area of environmental impact by depletion of resources (availability of resources): A nuclear energy system (NES) shall be capable of contributing to the energy needs in the twenty-first century while making efficient use of non-renewable resources* | ||
INPRO user requirements | Criteria | Indicator (IN) and Acceptance Limit (AL) |
---|---|---|
UR1: Consistency with resource availability:
The NES should be able to contribute to the world’s energy needs during the twenty-first century without running out of fissile/fertile material and other non-renewable materials, with account taken of reasonably expected uses of these materials external to the NES. In addition, the NES should make efficient use of non-renewable resources |
CR1.1: Fissile/fertile material | IN1.1: Quantity, Fj(t), of fissile/fertile material type j available for use in the NES at time t |
AL1.1: Fj(t) > Dj(t), quantity available for NES, Fj(t), should be bigger than quantity needed, Dj(t), for any t < 100 years | ||
CR1.2: Non-renewable materials | IN1.2: Quantity, Qi(t), of material type i available for use in the NES at time t | |
AL1.2: Qj(t) > Dj(t), quantity available for NES, Qj(t), should be bigger than quantity needed, Dj(t), for any t < 100 years | ||
CR1.3: Power supply to NES | IN1.3: P(t) = power available (from both internal and external sources) for use in the NES at time t | |
AL1.3: P(t) ≥ PNES(t), for any t < 100 years, where PNES(t) is the power required by the NES at time t | ||
CR1.4: End use of uranium | IN1.4: Ueu = end use (net) energy (GW·h) delivered by the NES per tonne of uranium mined | |
AL1.4: Ueu > U0; U0 = maximum achievable end use for an existing NES** with a once through (open) fuel cycle | ||
CR1.5: End use of thorium | IN1.5: Theu = end use (net) energy (GW·h) delivered by the NES per tonne of thorium mined | |
AL1.5: Theu > Th0; Th0 = maximum achievable end use for a current operating thorium cycle | ||
CR1.6: End use of non-renewable resources | IN1.6: Ci = end use (net) energy delivered by the NES per tonne of limited non-renewable resource i consumed | |
AL1.6: Ci > C0; C0 to be determined on a case specific basis | ||
UR2: Adequate net energy output:
The energy output of the NES should exceed the energy required to implement, operate and decommission the NES within an acceptably short period |
CR2.1: Amortization time | IN2.1: TEQ = time required to match the total energy input into the NES with energy output (years) |
AL2.1: TEQ << TL; TL = intended lifetime of NES |
* - Guidance provided here, describing good practices, represents expert opinion but does not constitute recommendations made on the basis of a consensus of Member States.
** - In the updated INPRO methodology, ‘existing NES’ means an ‘NES of latest design operating in 2013’.
Concept of sustainable development and its relationship with the area of environmental impact from depletion of resources of the INPRO methodology
The United Nations World Commission on Environment and Development Report[5] (often known as the Brundtland Report), entitled Our Common Future, defines sustainable development as: “development that meets the needs of the present without compromising the ability of future generations to meet their own needs” (see chapter 2, paragraph 1[5]). Moreover, this definition contains within it two key concepts:
- “the concept of ‘needs’, in particular the essential needs of the world’s poor, to which overriding priority should be given; and
- the idea of limitations imposed by the state of technology and social organization on the environment’s ability to meet present and future needs.”
This simple definition of sustainable development suggests a three part test for any approach to sustainability
and sustainable development: (i) current development should be fit for the purpose of meeting current needs with
minimized environmental impacts and acceptable economics; (ii) current RD&D programmes should establish
and maintain trends that lead to technological and institutional developments that serve as a platform for future
generations to meet their needs; and (iii) the approach to meeting current needs should not compromise the ability
of future generations to meet their needs.
At first reading, this definition may appear obvious, but when considering the complexities of implemented
nuclear energy technology and systems, plus their many supporting institutions, meeting the three part test is not
always straightforward because many approaches only meet one or perhaps two parts of the test in a given area,
and may fail on the others.
The Brundtland Report overview (para. 61[5]) of the topic of nuclear energy summarized that:
“After almost four decades of immense technological effort, nuclear energy has become widely used. During this period, however, the nature of its costs, risks, and benefits have become more evident and the subject of sharp controversy. Different countries world-wide take up different positions on the use of nuclear energy. The discussion in the Commission also reflected these different views and positions. Yet all agreed that the generation of nuclear power is only justifiable if there are solid solutions to the unsolved problems to which it gives rise. The highest priority should be accorded to research and development on environmentally sound and ecologically viable alternatives, as well as on means of increasing the safety of nuclear energy.”
The Brundtland Report presented its comments on nuclear energy in chapter 7, section III[5]. In the area of
nuclear energy, the focus of sustainability and sustainable development is on solving certain well known problems
(referred to here as ‘key issues’) of institutional and technological significance. Sustainable development implies
progress and solutions in the key issue areas. Seven key issues are discussed (in this order):
(a) Proliferation risks;
(b) Economics;
(c) Health and environment risks;
(d) Nuclear accident risks;
(e) Radioactive waste disposal;
(f) Sufficiency of national and international institutions (with particular emphasis on intergenerational and
transnational responsibilities);
(g) Public acceptability.
The INPRO methodology for the self-assessment of sustainability and sustainable development of a NES is
based on the broad philosophical outlines of the Brundtland Report’s concept of sustainable development described
above. Twenty-eight years have passed since the publication of the Brundtland Report, and 14 years have passed
since the initial consultancies on development of the INPRO methodology in 2001. In the interim period of time,
significant historical events have starkly highlighted certain key issues. However, the key issues for sustainable
development of NESs have remained essentially unchanged over nearly three decades.
By far the most notable events in the period, which have a direct impact on nuclear energy sustainability, are
related to non-proliferation, nuclear security, cost escalation of new construction and, most notably, the accident at
the Fukushima Daiichi nuclear power plant in 2011. The Fukushima Daiichi accident further clarified that nuclear
safety is an issue of paramount importance for sustainability and that external hazards, associated with a particular
site, could be responsible for a dramatic common cause failure involving multiple reactor units.
In each INPRO methodology manual, a key issue of NES sustainable development is examined. The structure
of the methodology is a hierarchy of INPRO BPs, URs and CR measuring whether the UR has been achieved.
Under each BP, the CR include measures that take into consideration the three part Brundtland Report’s sustainable
development test.
This INPRO manual focuses on the key issue of environmental impact through depletion of natural resources
associated with NES development and deployment. The Brundtland Report did not specifically identify depletion
of resources as a key issue in 1987. There are several reasons why these issues might have been omitted at the time,
which still exist today. These reasons are described below.
In a broad and general sense, NESs are frugal with respect to the use of natural resources when compared
to available dispatchable, baseload non-NESs. For example, it is unlikely that significant and sustainable pressure
will come to bear on natural uranium fuel resources until the second half of the current century or well beyond
that, depending on global nuclear energy growth and further discoveries of economically recoverable uranium
resources. When uranium fuel becomes sufficiently expensive, closed fuel cycles can produce many thousands of
years of nuclear fuel from existing fertile material resources. As the nuclear fuel cost is very low, taken as a fraction
of the nuclear electricity cost, significantly more expensive nuclear fuel is likely to be acceptable, depending upon
how a particular nuclear market is structured.
The more complex sustainability questions in the area of resource depletion pertain to use of other mineral
resources that are rarer than nuclear fuels (e.g. critical minor constituents in metal alloys and other materials that are
particular to nuclear energy technology). However, if a country decides that its NES should depend wholly upon its
own mineral resources, rather than the international market, in some cases, this may also imply more severe limits
to nuclear fuel sustainability. Questions about when and how diversification from a uranium fuel economy should
expand to include a growing share of other nuclear fuels are more associated with development of roadmaps for
reactor and fuel cycle technology development and deployment than they are with ultimate resource depletion. Even
so, that progress is being made (or is planned) to achieve more effective fuel utilization is important, particularly
for time periods extending well into the second half of this century. Essentially, indefinite and irretrievable direct
geological disposal of once through uranium and thorium fuels may imply an unsustainable NES in the longer
term. It also may also damage future nuclear fuel resource economics to a degree that it impugns nuclear energy as
an option available to future generations, thus failing a key Brundtland Report test of sustainability.
This INPRO manual tests whether or not an NES is sustainable with respect to fissile/fertile and non-renewable
material resources within a period of a century. It also tests whether or not greater net end use energy is being
(or planned to be) produced from mined uranium and thorium than the maximum achievable under current open
fuel cycles. Taken together, these measures directly address the question of whether NESs, implemented to meet
current needs, are having minimum intergenerational impact from the perspective of natural resource depletion,
thus satisfying the three part Brundtland Report sustainable development test.
General features and necessary INPUT for an assessment
This section provides some general background information on environmental issues, particularly on the environmental impact from depletion of resources caused by an NES. The necessary input and its sources for an assessment of an NES in the INPRO methodology area of environmental impact from depletion of resources are also defined.
Concept of sustainable development
The concept of sustainability can be considered from several related, but different, points of view:
social, economic, environmental and institutional. This publication deals with the environmental dimension of
sustainability, by considering issues related to depletion of natural resources.
Protection of the environment is a major consideration in the processes for approving industrial activities in
many countries. The level of international societal concern for the environment is clearly indicated in publications
reflecting international consensus, notably the Brundtland Report[5], the Rio Declaration on sustainable
development[6] and the Joint Safety Convention of the IAEA[7].
The common basic idea in these publications is that the present generation should not compromise the ability
of future generations to fulfil their needs and should leave them with a healthy environment. Nuclear power should
support sustainable development by providing much needed energy with relatively low burdens on the atmosphere,
water, land and resource use. Efficient and effective use of resources will be necessary. Moreover, improvement
of the technology should include improvement of its environmental aspects to a degree that is consistent with
importance to society and with the potential environmental performance of competing technologies.
Interfaces of a nuclear energy system with the environment
The different components or facilities of a complete NES are presented in Fig. 1, starting with mining and processing, through to the final disposal of nuclear waste.
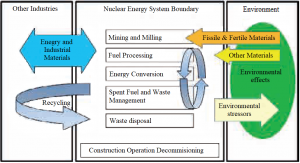
An NES has several interfaces with the environment and other industries. From the environment,
non-renewable resources such as fissile and fertile materials, e.g. uranium and thorium (orange arrow in Fig. 1), are
removed and used in the NES together with other materials such as zirconium (bright yellow arrow in Fig. 1). On
the other hand, the NES produces some stressors, e.g. release of radioactive nuclides, that have an adverse impact
on the environment (pale yellow arrow in Fig. 1).
In addition to these environmental effects, an NES is exchanging with other industries the energy and
industrial materials required for the installation, operation and finally decommissioning of the nuclear facilities
(blue arrow in Fig. 1).
Ideally, an environmental impact assessment of an NES takes all these interfaces into account during the
lifetime of the system.
Specification of the nuclear energy system
A prerequisite for an INPRO assessment is the specification of the NES (see overview of the INPRO
methodology) to be assessed. The NES is to be defined by the INPRO assessor.
For an environmental assessment, in principle, the following aspects should be covered in the specification
of the NES:
- The complete NES should be considered, covering the entire front end of the fuel cycle, the energy conversion unit (reactor) and the entire back end comprising waste repositories. For all nuclear facilities, the complete lifetime should be covered, i.e. construction, operation and decommissioning.
- If necessary, environmental burdens may be divided into national (or regional) burdens and those occurring outside the country (or regional) borders, if such information would be required by stakeholders.
Thus, by meeting the above defined requirements, the general underlying principle of assessing the entirety
of the environmental impacts would not be violated.
However, in general, cut-offs, i.e. selection of specific facilities of an NES, will be required in an INPRO
assessment. Such an approach is recommended for nuclear technology users and specifically in the case that a
country is embarking on a nuclear power programme, i.e. the INPRO environmental assessment in such a country
should cover only the first nuclear power plant and related waste management facilities. A nuclear technology
developer may focus the environmental assessment (analysis) on their design of a nuclear facility under
development.
Information on demand and supply of resources
To assess criterion CR1.1 of UR1, several generic studies are available that define the global demand and the
availability of primary (and secondary, if a global market exists for it) supply of natural uranium (and other fissile
materials) needed for nuclear facilities within the next 100 years. These studies, presented in Appendices I–IV,
cover different development rates of demand and different fuel cycles.
To assess criterion CR1.2 of UR1, the results of a generic study for global demand and supply of other key
materials in an NES are needed to complete an INPRO assessment. A recently performed generic study on global
supply and demand of non-renewable materials is summarized in Appendix II, and could be used as reference in
the assessment.
To assess criterion CR1.3 of UR1, information about the power needed to construct, operate and decommission
a typical nuclear power plant currently operating is available, but for the NES assessed, this information is to be
provided by the (potential) supplier.
To assess criterion CR1.4 and criterion CR1.5 of UR1, the net energy delivered by the NES assessed per
tonne of uranium and thorium used is to be calculated. For uranium, this value can be determined by the INPRO
assessor using a simplified tool, if the assessor has access to the specific characteristics of the assessed NES, such
as the thermal efficiency and core average burnup of the nuclear power plant to be installed in the country.
To assess criterion CR1.6 of UR1, the end use of key materials (other than fissile and fertile materials) needed
in the NES assessed and in the current NES is to be determined. For such a study, close cooperation between the
assessor and the (potential) supplier is necessary. For some key materials, the results of generic studies on a current
NES are available.
To assess criterion CR2.1 of UR2, a reference study is available that defines the energy payback time (EPBT)
for a typical currently operating NES.
Other sources of INPUT
A comprehensive INPRO assessment of the planned NES in the Republic of Belarus has been performed between 2009 and 2011, and is documented in Ref.[9].
INPRO Basic Principle, User Requirement and Criteria
This section presents the BP, the URs and the CR in the INPRO methodology area of environmental impact caused from depletion of resources.
INPRO Basic Principle: availability of resources
INPRO basic principle: The NES shall be capable of contributing to the energy needs in the twenty-first century
while making efficient use of non-renewable resources.
To be environmentally acceptable, the NES assessed must be sustainable and not run out of important
resources part way through its intended lifetime. These resources include fissile/fertile materials, water (when
supplies are limited or quality is under stress) and other critical materials. The NES should also use them at least as
efficiently as acceptable alternatives, both nuclear and non-nuclear. Even in the absence of a viable alternative, the
best use possible is to be made of non-renewable resources.
Sustainability of an NES requires primarily that it fits its purpose. This has different aspects, which are
addressed separately within the different INPRO methodology areas (e.g. economics, safety). All aspects should
be considered, and the designer and operator of the system should strive to achieve optimal conditions with
minimal effects on the environment, for which compromises across the areas may be necessary. Thus, the INPRO
methodology requires a holistic approach, i.e. the whole NES should be considered in all areas, and all material
flows in and out of the NES should be accounted for, including resources taken from, as well as stressors emitted
to, the environment.
The INPRO methodology has defined two URs, UR1 and UR2, for the BP.
User requirement UR1: consistency with resource availability
User requirement UR1: The NES should be able to contribute to the world’s energy needs during the twenty-first
century without running out of fissile/fertile material and other non-renewable materials, with account taken of
reasonably expected uses of these materials external to the NES. In addition, the NES should make efficient use of
non-renewable resources.
UR1 addresses continuous availability and consumption of non-renewable resources. Primarily, it should be
demonstrated that the NES assessed will operate throughout the twenty-first century without incurring fuel shortages
and lack of strategic materials. The time horizon of about 100 years was chosen in the INPRO methodology based
on the consideration that beyond this period, uncertainties become too large in any evaluation result.
To demonstrate that this UR is met, careful consideration should be given to the implications of available
resources with appropriate choice of the boundary of the NES assessed (see Fig. 1). The availability and
consumption of resources generally require a global, rather than an individual national or regional, evaluation.
In addition, resources considered, especially those of fissile/fertile materials, need to include estimated resources
beyond those currently fully proven. When addressing unconventional sources (e.g. uranium coextraction with
phosphates or extraction of uranium from sea water (see Appendix I for more information)), the assessor should
bear in mind that such unconventional resources of fissile/fertile materials may have implications not only on the
environment, but also on the costs of nuclear fuel.
Global, regional and national energy demand/supply scenarios
To address UR1, it needs, in principle, to be demonstrated that the NES demand for non-renewable resources
can be supplied at any time that the NES is operated during the twenty-first century, considering the entire lifetime
(commissioning, operation and decommissioning) of all its facilities. As a global market exists and is likely to
continue existing for all NES related non-renewable resources, such a demonstration generally requires a global
analysis that is beyond the reasonably expected capability of any individual country performing an NES assessment.
The way forwards here may be twofold.
One way is to borrow from the results of the recently completed international studies on global availability of
non-renewable resources; in order to facilitate this method, Appendices I–IV are included in the current publication,
where the main results of such studies and the approaches used are highlighted and summarized, complete with a
list of references to full reports on these studies.
On the other hand, if a country performing the NES assessment has great plans for its nuclear energy
programme and foresees it could eventually become a major player in global nuclear energy markets, then it makes
sense to consider joining efforts with other countries to perform an updated global resource availability assessment.
Such assessments are being periodically undertaken under the aegis of renowned international organizations, and
one option to do so is to join the activities of the IAEA/INPRO task ‘Global scenarios’.
Notwithstanding the existence of a global market for NES related non-renewable resources, national
assessment also makes sense once the country considers ensuring that its national nuclear power programme
benefits from its own domestic resources. Assessment of this kind will be useful for understanding the national
resource base and national economy potential, if only in the medium and long terms, but it will only partially
support the assessment against the UR1 requirement.
Regional assessment could be recommended in the case of existing or foreseen long term partnerships with
particular neighbouring or non-neighbouring countries with which good relations and cooperation in the nuclear
energy field exist. Such an assessment could foster further cooperation, potentially resulting in a sustainable
regional NES; however, as in the case of a national assessment, it would only partially support the assessment
against UR1.
In both national and regional assessments, the missing ‘rest of the world’ information could be retrieved from
the already completed resource availability studies such as those presented in the appendices to this publication.
Summarized below are the factors that are important for non-renewable resource availability assessment of
relevance to UR1.
For an INPRO assessment of the environmental impact from depletion of resources, the global and national
(regional) scenarios of nuclear capacity growth first need to be specified, including data on time dependent capacity
additions and corresponding reactor types. To obtain an idea of possible global scenarios, the information presented
in Refs[2][3] may be useful. These studies provide a range of plausible (and even some idealistic) global nuclear
energy capacity increases, and following several scenarios within the range may aid in understanding how the
existing large uncertainties could affect the results of a UR1 INPRO assessment.
Also useful could be an analytical framework for material flow analyses developed within the collaborative
project GAINS. This framework, presented in Ref.[2], includes internationally verified data for many existing and
future reactors, descriptions of closed fuel cycles, storylines and scenarios of global nuclear energy development
up to the end of this century, results of cross-verification and recommendations on the use of material flow analysis
codes, and other relevant information.
The INPRO methodology defines six CR (CR1.1–CR1.6) for UR1, as shown in Table 1.
Criterion CR1.1: Fissile/fertile material
Indicator IN1.1: Fj(t), quantity of fissile/fertile material of type j available for use in the NES at time t.
Acceptance limit AL1.1: Fj(t) > Dj(t), quantity available for NES, Fj(t), should be bigger than quantity needed,
Dj(t), for any t < 100 years.
Types of resources of fissile and fertile materials
In order for an NES to operate successfully, i.e. to contribute to satisfying the world’s energy needs during
the twenty-first century without running out of resources, UR1 must be primarily applied to fissile/fertile materials,
i.e. compliance must be demonstrated with CR1.1, confirming that the quantity of fissile/fertile material type j
available at time t will always be more than needed in the NES assessed at any time t during a period of 100 years.
A fissile material is one that is capable of sustaining a chain reaction of nuclear fission. The principal fissile
materials are 235U (0.7% of naturally occurring uranium), 239Pu, 241Pu and 233U, with the last three being artificially
produced from the fertile materials 238U and 232Th. A fertile material, not itself capable of undergoing fission by
neutrons, is one that decays into fissile material after neutron absorption. Fertile materials are 232Th, which can be
converted into fissionable 233U, and 238U, which can be converted into fissionable 239Pu.
In general, two kinds of sources of fissile/fertile materials are distinguished: primary resources and secondary
supply; the latter is also sometimes called the secondary source. Estimations of the availability of such resources
can be found in a diverse set of publications by national and international organizations. Table 2 gives an overview
of types of resources of fissile and fertile materials, and the references from which information can be retrieved.
Resources of fissile/fertile materials | References |
---|---|
Primary resources* | |
Natural uranium
Natural thorium |
Appendix I; Refs[10][11] |
Secondary supply | |
Depleted uranium (including its re-enrichment) Natural uranium inventory (governmental and commercial) drawdown Highly enriched uranium available for down blending (with depleted uranium essentially) |
Appendix I; Ref.[11] |
Reprocessed uranium[11][12] | |
Plutonium from reprocessing of civil spent uranium fuel Plutonium from the surplus military Pu stock Spent nuclear fuel available for reprocessing Transuranium elements in spent fuel retrievable for later use Separated minor actinides (Np, Cm and Am) stock Uranium-238 produced by reprocessing of Th fuel |
National data**; |
* - These primary resources are still to be mined, i.e. they are not yet recovered from their natural environment, but due account is taken of the losses that mining would entail, i.e. these resources represent the net available resources that would be available after mining.
** - ‘National data’ indicates that no international referenced data are available in the public domain.
The availability of fissile/fertile materials should be considered according to different scales. As has already
been mentioned, because the primary resources of uranium and thorium are available via a global market, the
availability of primary resources needs to be considered in an INPRO assessment on a global scale. However, most
of the secondary supply is available on a national (or regional) scale only, and can, therefore, be considered only by
individual Member States in national or regional assessments.
Of the secondary uranium sources, depleted uranium produced at enrichment facilities, natural uranium
withdrawn from inventories held by utilities and governments, and separated irradiated uranium (reprocessed
uranium (REPU)) produced in reprocessing facilities could be used in (partly) closed nuclear fuel cycles and are
also available on a global market because of the ease of transportation of these materials.
Transuranics (TRUs), i.e. plutonium, and minor actinides (MAs) resulting from reprocessing of civil spent
uranium based fuel, are today part of national NESs with a (partly) closed nuclear fuel cycle and are currently
absent from any global market mechanism trading these materials. The possible transfer of such TRUs from outside
into an NES has been taken into account in studies presented in Appendices II and III, looking very far into the
future, i.e. covering a time period until the end of the century. The same applies to the spent fuel amount and the
TRUs contained in that spent fuel.
Plutonium originating from surplus military stocks should be considered on a national scale, because of the
limited availability of this resource based on bilateral contracts between certain Member States.
Thorium resources
There is still less known about the primary thorium resources compared to the uranium resources. Large
thorium resources are found in Australia, Brazil, Canada, China, Egypt, India, Norway, Russian Federation, South
Africa, Turkey, United States of America (USA) and the Bolivarian Republic of Venezuela. Existing estimates of
thorium resources total more than 6.5 Mt Th. These estimates are considered conservative because the historically
weak demand has limited thorium exploration[13].
Classification of primary resources according to their recoverability defined by the IAEA and the OECD
Nuclear Energy Agency (OECD/NEA) is described in Appendix I.
Secondary uranium supply
It is generally expected that the role of global secondary uranium supply will diminish during the coming
decades due to drawdown of natural uranium inventories and the limited availability of military plutonium to be
used in a limited number of reactors. Currently, the main global secondary supply is based on down blending
of highly enriched uranium (HEU), and in the medium term, re-enrichment of depleted uranium as long as the
economic balance of this option versus virgin uranium mining remains attractive.
In case the NES assessed by an INPRO assessor might not be installed before the year 2030 or later, the
impact of global secondary supply is likely to be limited. Therefore, in such a case, the INPRO assessment may not
need to take into account the global secondary supply, except for, possibly, the re-enrichment of depleted uranium,
depending on its economic viability and the availability of enrichment capacity. Above all, if nuclear energy is to
remain an option for the longer term, renewal of exploration and opening of new mineable uranium resources have
to be relaunched in order to supply most and soon all of the uranium requirements for the global nuclear reactor
fleet.
Balance of demand and available resources of fissile/fertile materials
Criterion CR1.1 demands two considerations:
- Knowledge of the amount of fissile/fertile material available for the NES assessed. These available resources consist of global and national (regional) resources of fissile and fertile materials, including plutonium in the case of a closed fuel cycle, or 233U in a fuel cycle using thorium as the fertile material.
- Knowledge of the amount of fissile/fertile material needed in the NES assessed. This demand for resources consists of fissile/fertile materials entering the NES, i.e. being supplied as reload fuel.
Estimation of available amount of global primary uranium resources
Appendix I presents the status of available fissile and fertile materials based on the ‘Red Book’, a joint report
by the IAEA and OECD/NEA[13]. As of 2011, there were ~12 × 106 tonnes of natural uranium (tU) available
in the cost category of <US $130, and ~18 × 106 tU in the cost category of <US $260, defined as conventional
resources. In addition to these conventional resources, there are estimated resources of the same order of magnitude
defined as unconventional uranium resources that include uranium found in phosphates and black shale, with
estimated prices slightly above the ones for conventional resources.
Uranium contained in sea water would be available in an amount greater than 4 × 109 tU, although at a
significantly higher price. Appendix I emphasizes the fact that the uranium supply is based on a global free market
that reacts appropriately to higher prices because of expected shortages by increased exploration and production
capacity.
Long term perspective of global demand for primary resources of fissile and fertile materials
In Appendix I, which contains a summary of the 2011 edition of the Red Book[13], the demand for natural
uranium for the global NES up to 2035 is estimated. In Appendix III, a summary of a more recent comprehensive
study regarding global demand for primary fissile/fertile resources until the end of the twenty-first century is
presented, which takes into account several parameters such as different types of fuel cycles, different types of
reactors, different rates of installation of nuclear facilities, etc. This generic study and the analytical framework
developed within it can be used as a basis for all INPRO assessments of the environmental impact from depletion
of resources.
Estimation of available amount of national (regional) primary resources of fissile and fertile materials
These data should be available in the country (countries) where the NES assessed is (to be) installed.
The INPRO assessor should refer to publications of responsible national (or regional) organizations to get this
input. Alternatively, the INPRO assessor can use some publications from international organizations such
as the OECD/NEA–IAEA Red Book[13] and the IAEA Integrated Nuclear Fuel Cycle Information System
(INFCIS)[10] that also list these resources based on a national estimation.
Long term perspective of national (regional) demand for resources of fissile and fertile materials
This input should be available to the INPRO assessor for the country (countries) where the NES is (to be)
located. The NES demand for resources depends on the national (regional) scenario (e.g. rate of installation and
types of nuclear facilities) of nuclear power introduction (or expansion), and, thus, the establishment of a national
(regional) nuclear power scenario is a prerequisite to preparing this input (i.e. creation of such a national nuclear
scenario is a prerequisite for an INPRO assessment).
To determine the demand for resources for the NES assessed, the INPRO assessor should receive information
from (potential) technology suppliers (how many primary resources (e.g. tonnes of natural uranium) are needed for
each nuclear facility), especially for nuclear reactor(s), e.g. tU per GW(e)·a.
If fissile/fertile materials are not recycled in the NES assessed (e.g. open or once through fuel cycle),
estimation of the total (lifetime) uranium demand for the NES assessed is rather straightforward, namely, just the
integration of demand of the primary resource per year over the projected lifetime of the system. Usually, this value
should have been already determined in an energy system planning study that is, by definition, a prerequisite for an
INPRO assessment (see overview of the INPRO methodology).
If resources are (to be) recycled within the NES assessed (e.g. an NES with a partly or completely closed fuel
cycle), information is needed on how much fissile/fertile materials are recovered from spent fuel, which could be
used as a secondary supply for the NES. Some of this information needed is design specific, e.g. the correlation
between the nuclide composition of fresh fuel and spent fuel in a reactor, which depends on core design including
the neutron spectrum, fuel arrangement (e.g. seed and blanket arrangements), burnup, etc. To determine the amount
of recycled fissile/fertile materials, the results of more sophisticated tools for material flow analysis, such as the
Nuclear Fuel Cycle Simulation System (NFCSS)[10] or the Model for Energy Supply Strategy Alternatives and
their General Environmental Impact (MESSAGE), are necessary; these are available on request from the IAEA.
Final assessment of CR1.1: Fissile/fertile material
Global demand and supply of fissile/fertile materials
A detailed study performed within the INPRO project — summarized in Appendix III — came to the
conclusion that the currently identified global resources of natural uranium that have a high probability of being
provided at reasonably low cost are sufficient to supply fuel to a global NES (consisting of thermal reactors) whose
installed capacity could increase to 2500 GW(e) by the end of the century, which is approximately six times greater
than the current installed capacity of less than 400 GW(e). The results presented in the Red Book 2011[13] —
summarized in Appendix I — confirm this trend up to the year 2035, and predict that this trend will continue during
this century. If the deployment of fast reactors (FRs) is realized in a sufficient amount, which appears likely at the
moment in view of developments in a number of technology holder countries worldwide, the currently identified
uranium resources (~18 × 106 tU) would be capable of supporting the growth of a global NES with a capacity that
is 12 times larger than that of today at the end of the century, i.e. with a capacity of 5000 GW(e). Thus, the balance
of global demand and supply of natural uranium has been confirmed until the end of the century in this INPRO
study.
INPRO assessors are asked to study the full reports summarized in Appendices I and III, familiarize
themselves with the results, and document the main conclusions thereof in the final assessment report. It is also
recommended that they contact the IAEA INPRO section, which has an ongoing project on global nuclear energy
scenarios. If new studies on this issue become available, they should also be taken into account by the INPRO
assessors.
National (regional) demand and supply of fissile/fertile materials
As the global resources of uranium have been found to be sufficient, it can also be concluded that each
national NES will have access to sufficient uranium, as long as a global free market for natural uranium supply
(and/or nuclear fuel) prevails. National or regional assessments could then be performed to understand how the
country, in its national nuclear power programme, could benefit from its own domestic resources and how regional
cooperation could potentially be fostered (see the discussion at the beginning of Section 3.2). For this purpose, it is
recommended that the INPRO assessor determine the uranium resources necessary for the national (regional) NES
assessed using one of the tools available from the IAEA (MESSAGE, NFCSS and Dynamic Energy System —
Atomic Energy (DESAE)). To confirm the availability of the required resources, the assessor should take existing
national (regional) resources into account.
Criterion CR1.2: Non-renewable materials
ᅠIndicator IN1.2: Quantity, Qi(t), of material type i available for use in the NES at time t.ᅠ
|
---|
Acceptance limit AL1.2: Qj(t) > Dj(t), quantity available for NES, Qj(t), should be bigger than quantity needed, Dj(t), for any t < 100 years. |
Criterion CR1.3: Power supply to the nuclear energy system
ᅠIndicator IN1.3: P(t) = power available (from both internal and external sources) for use in the NES at time t.ᅠ
|
---|
Acceptance limit AL1.3: P(t) ≥ PNES(t) for all t < 100 years, where PNES(t) is the power required by the NES at time t.
Regarding power supplied to NESs based on fossil fuel energy, several references exist on fossil fuel resources that can be used for the assessment, e.g. Refs[16][17]. Scenarios for fossil fuel exploitation and use are described in these two references when discussing uranium scenarios. |
Criterion CR1.4: End use of uranium
ᅠIndicator N1.4: Ueu = end use (net) energy (GW·h) delivered by the NES per tonne of uranium mined.ᅠ
|
---|
Acceptance limit AL1.4: Ueu > U0; U0 = maximum end use achievable for an existing NES with a once through nuclear fuel cycle. |
Criterion CR1.5: End use of thorium
ᅠIndicator IN1.5: Theu = end use (net) energy (GW·h) delivered by the NES per tonne of thorium mined.ᅠ
|
---|
Acceptance limit AL1.5: Theu > Th0; Th0 = maximum end use achievable with a current operating thorium cycle. |
Criterion CR1.6: End use of other non-renewable resources
ᅠIndicator IN1.6: Ci = end use (net) energy delivered by the NES per tonne of limited non-renewable resource consumed.ᅠ
|
---|
Acceptance limit AL1.6: Ci > C0; C0 to be determined on a case specific basis. |
Material | Minimum (GW·h/t) | Maximum (GW·h/t) |
---|---|---|
Copper | 1.68 × 102 | 2.07 × 102 |
Iron | 2.93 | 3.66 |
Gravel | 2.44 × 10-1 | 3.06 × 10-1 |
User requirement UR2: adequate net energy output
User requirement UR2: The energy output of the NES should exceed the energy required to implement, operate and
decommission the NES within an acceptably short period.
The net energy output of an NES is the usable energy produced by the system over and above the energy
required to install, operate and decommission the system, over its intended life cycle. The net energy balance
(output minus input) should turn to a positive value in an acceptably short period after startup; obviously, the
shorter the better.
The INPRO methodology has defined one criterion for UR2, as shown in Table 1.
Criterion CR2.1: Amortization time
ᅠIndicator IN2.1: TEQ = time required to match the total energy input into the NES with energy output (years).ᅠ
|
---|
Acceptance limit AL2.1: TEQ << TL; TL = intended lifetime of NES. |
Appendixes
[ + ] Assessment Methodology | |||||
---|---|---|---|---|---|
|
References
- ↑ INTERNATIONAL ATOMIC ENERGY AGENCY, INPRO Methodology for Sustainability Assessment of Nuclear Energy Systems: Environmental Impact of Stressors, IAEA Nuclear Energy Series No. NG-T-3.14, IAEA, Vienna (in preparation).
- ↑ 2.0 2.1 2.2 INTERNATIONAL ATOMIC ENERGY AGENCY, Framework for Assessing Dynamic Nuclear Energy Systems for Sustainability: Final Report of the INPRO Collaborative Project GAINS, IAEA Nuclear Energy Series No. NP-T-1.14, IAEA, Vienna (2013).
- ↑ 3.0 3.1 3.2 INTERNATIONAL ATOMIC ENERGY AGENCY, Nuclear Energy Development in the 21st Century: Global Scenarios and Regional Trends, IAEA Nuclear Energy Series No. NP-T-1.8, IAEA, Vienna (2010).
- ↑ INTERNATIONAL ATOMIC ENERGY AGENCY, INPRO Methodology for Sustainability Assessment of Nuclear Energy Systems: Economics, IAEA Nuclear Energy Series No. NG-T-4.4, IAEA, Vienna (2014).
- ↑ 5.0 5.1 5.2 5.3 5.4 UNITED NATIONS, Our Common Future, Report of the World Commission on Environment and Development, UN, New York (1987), [1]; [2]
- ↑ UNITED NATIONS, Conference on Environment and Development, Vol. I, Resolutions Adopted by the Conference (UN publication, Sales No. E.93.I.8), Rio de Janeiro (1992).
- ↑ INTERNATIONAL ATOMIC ENERGY AGENCY, Joint Convention on the Safety of Spent Fuel Management and on the Safety of Radioactive Waste Management, INFCIRC/546, IAEA, Vienna (1997).
- ↑ INTERNATIONAL ATOMIC ENERGY AGENCY, Guidance for the Application of an Assessment Methodology for Innovative Nuclear Energy Systems, INPRO Manual: Environment, Vol. 7, Final Report of Phase 1 of the International Project on Innovative Nuclear Reactors and Fuel Cycles (INPRO), IAEA-TECDOC-1575/Rev.1, IAEA, Vienna (2008).
- ↑ INTERNATIONAL ATOMIC ENERGY AGENCY, INPRO Assessment of the Planned Nuclear Energy System of Belarus, Report of the International Project on Innovative Nuclear Reactors and Fuel Cycles (INPRO), IAEA-TECDOC-1716, IAEA, Vienna (2013).
- ↑ 10.0 10.1 10.2 INTERNATIONAL ATOMIC ENERGY AGENCY, Integrated Nuclear Fuel Cycle Information System (INFCIS), IAEA, Vienna (2012), [3]
- ↑ 11.0 11.1 11.2 INTERNATIONAL ATOMIC ENERGY AGENCY, Analysis of Uranium Supply to 2050, IAEA, Vienna (2001).
- ↑ INTERNATIONAL ATOMIC ENERGY AGENCY, Use of Reprocessed Uranium: Challenges and Options, IAEA Nuclear Energy Series No. NF-T-4.4, IAEA, Vienna (2009).
- ↑ 13.0 13.1 13.2 13.3 13.4 ORGANISATION FOR ECONOMIC CO-OPERATION AND DEVELOPMENT NUCLEAR ENERGY AGENCY, INTERNATIONAL ATOMIC ENERGY AGENCY, Uranium 2011: Resources, Production and Demand, (‘Red Book’), OECD/NEA, Paris (2012).
- ↑ 14.0 14.1 14.2 UNITED STATES GEOLOGICAL SURVEY, Mineral Commodity Summaries, USGS, US Department of the Interior (2013), [4]
- ↑ 15.0 15.1 15.2 15.3 15.4 ORGANISATION FOR ECONOMIC CO-OPERATION AND DEVELOPMENT NUCLEAR ENERGY AGENCY, A Preliminary Assessment of Raw Material Inputs that would be Required for Rapid Growth in Nuclear Generating Capacity, OECD/NEA, Paris (2011).
- ↑ BRITISH PETROLEUM, BP Statistical Review of World Energy 2015, BP, London (2015), [5]
- ↑ WORLD ENERGY COUNCIL, World Energy Resources: 2013 Survey, WEC, London (2013), [6]
- ↑ 18.0 18.1 18.2 18.3 18.4 DONES, R., “Kernenergie”, Sachbilanzen von Energiesystemen: Grundlagen für den ökologischen Vergleich von Energiesystemen und den Einbezug von Energiesystemen in Öko-bilanzen für die Schweiz, Paul Scherrer Institut, Villigen, Swiss Centre for Life Cycle Inventories, Dübendorf (2003)
- ↑ 19.0 19.1 DONES, R., HECK, T., FAIST EMMENEGGER, M., JUNGBLUTH, N., Life cycle inventories for the nuclear and natural gas energy systems, and examples of uncertainty analysis, Int. J. Life Cycle Assess. 10 1 (2005) 10.
- ↑ ECOINVENT, Database, Centre for Life Cycle Inventories, [7]
- ↑ INTERNATIONAL ATOMIC ENERGY AGENCY, Lessons Learned from Nuclear Energy Systems Assessments (NESA) Using the INPRO Methodology. A report of the International Project on Innovative Nuclear Reactors and Fuel Cycles (INPRO), IAEA-TECDOC-1636, IAEA, Vienna (2009).
- ↑ UNITED STATES DEPARTMENT OF THE INTERIOR, US Geological Survey, 2011 Minerals Yearbook, Zirconium and Hafnium [Advance Release] (2013).
- ↑ NUCLEAR REGULATORY COMMISSION, Certificate of Compliance for Radioactive Materials Packages, Certificate No. 9261, US NRC, Washington, DC (2000), [8]
- ↑ FRISCHKNECHT, R., et al., Overview and Methodology, Ecoinvent Report No. 1, Swiss Centre for Life Cycle Inventories, Dübendorf (2007), [9]
- ↑ WORLD NUCLEAR ASSOCIATION, The Global Nuclear Fuel Market, Supply and Demand 2003–2025, WNA, London (2003).